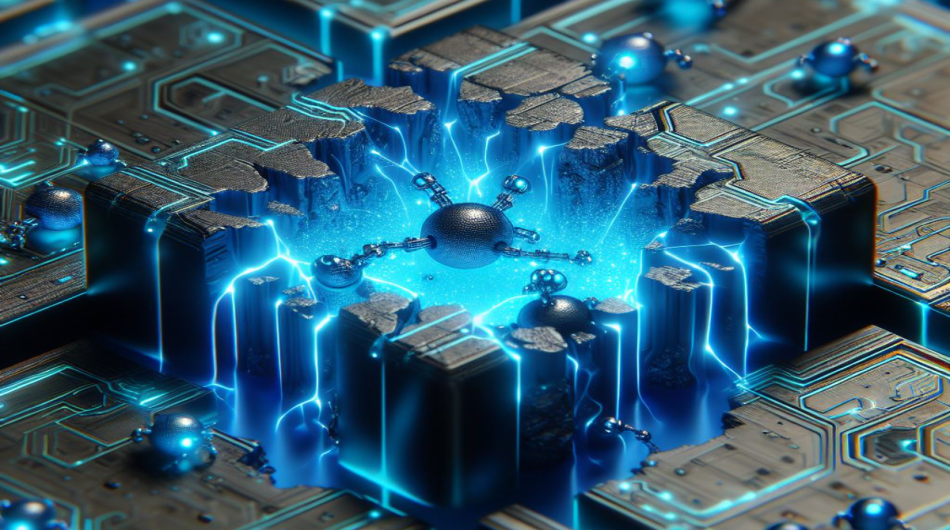
Self-healing materials are a class of smart materials that have the ability to repair themselves autonomously or with minimal external intervention when damaged. These materials have the potential to revolutionize various industries, from aerospace and automotive to construction and electronics, by increasing the durability, reliability, and lifespan of products and structures. This article provides a comprehensive overview of self-healing materials, including their principles, types, applications, challenges, and future prospects.
Principles of Self-Healing
The concept of self-healing in materials is inspired by biological systems, such as the human skin, which can heal itself after injury through a complex process involving inflammation, cell proliferation, and tissue remodeling. In the context of artificial materials, self-healing can be achieved through various mechanisms, depending on the type of material and the nature of the damage.
Autonomic vs. Non-Autonomic Healing
Self-healing materials can be classified into two main categories based on their healing mechanism: autonomic and non-autonomic.
Autonomic self-healing materials can repair themselves without any external intervention, such as heat or light. These materials typically contain a healing agent, such as a catalyst or a monomer, that is released upon damage and initiates the healing process. The healing agent may be encapsulated in microcapsules or embedded in a vascular network within the material.
Non-autonomic self-healing materials, on the other hand, require some form of external stimulus, such as heat, light, or pressure, to activate the healing process. These materials may contain reversible bonds, such as hydrogen bonds or ionic interactions, that can break and reform under certain conditions.
Intrinsic vs. Extrinsic Healing
Self-healing materials can also be classified based on whether the healing mechanism is intrinsic or extrinsic to the material.
Intrinsic self-healing materials have a healing capability that is inherent to the material itself, without the need for any additional components. These materials typically rely on reversible chemical bonds or physical interactions that can break and reform under certain conditions, such as temperature or pH changes. Examples of intrinsic self-healing materials include ionomers, supramolecular polymers, and shape memory polymers.
Extrinsic self-healing materials, on the other hand, rely on external components, such as microcapsules or vascular networks, to deliver the healing agent to the damage site. These materials typically require a higher level of complexity in their design and fabrication, but they can provide a more robust and efficient healing capability.
Healing Efficiency and Repeatability
The performance of self-healing materials can be evaluated based on two main criteria: healing efficiency and repeatability.
Healing efficiency refers to the extent to which the material can recover its original properties, such as strength or conductivity, after damage. This can be quantified by comparing the properties of the healed material to those of the pristine material, and is typically expressed as a percentage.
Repeatability refers to the ability of the material to undergo multiple healing cycles without significant degradation in its properties. This is an important consideration for applications where the material may be subjected to repeated damage, such as in flexible electronics or structural composites.
The healing efficiency and repeatability of self-healing materials depend on various factors, such as the type and extent of damage, the healing mechanism, the environmental conditions, and the material composition. Optimizing these factors is a key challenge in the development of self-healing materials, as discussed in later sections of this article.
Types of Self-Healing Materials
Self-healing materials can be categorized based on their composition and healing mechanism. Some of the most common types of self-healing materials include:
Polymers
Self-healing polymers are the most extensively studied and widely used type of self-healing materials. These materials can be further classified into several subtypes based on their healing mechanism:
Capsule-Based Self-Healing Polymers
Capsule-based self-healing polymers contain microcapsules filled with a healing agent, such as a monomer or a catalyst, that is released upon damage. The healing agent then polymerizes or cross-links with the surrounding matrix, filling the crack and restoring the material's properties. The microcapsules can be made of various materials, such as urea-formaldehyde or melamine-formaldehyde, and can be dispersed throughout the polymer matrix using techniques such as in-situ polymerization or solvent evaporation.
Vascular Self-Healing Polymers
Vascular self-healing polymers contain a network of channels or fibers that deliver the healing agent to the damage site. The channels can be created using various methods, such as 3D printing, sacrificial templating, or electrospinning. The healing agent can be stored in an external reservoir or integrated into the vascular network itself. Vascular self-healing polymers can provide a more efficient and repeatable healing capability compared to capsule-based systems, but they are also more complex and challenging to fabricate.
Intrinsic Self-Healing Polymers
Intrinsic self-healing polymers rely on reversible chemical bonds or physical interactions that can break and reform under certain conditions, such as temperature or pH changes. Examples of intrinsic self-healing polymers include:
-
Ionomers: Polymers containing ionic groups that can form reversible ionic clusters, providing a self-healing capability through ionic interactions.
-
Supramolecular polymers: Polymers containing non-covalent bonds, such as hydrogen bonds or π-π stacking, that can break and reform reversibly, enabling self-healing.
-
Shape memory polymers: Polymers that can recover their original shape after deformation, providing a self-healing capability through the release of stored elastic energy.
Metals
Self-healing metals are a relatively new class of materials that can repair cracks or damage through various mechanisms, such as precipitation, segregation, or phase transformation. Some examples of self-healing metals include:
-
Precipitation-based self-healing metals: Metals that can form precipitates, such as carbides or nitrides, at the crack surface, filling the crack and preventing its propagation.
-
Segregation-based self-healing metals: Metals that can segregate certain elements, such as boron or carbon, to the grain boundaries, enhancing the cohesive strength and resistance to crack propagation.
-
Shape memory alloys: Metals that can recover their original shape after deformation, providing a self-healing capability through the release of stored elastic energy.
Ceramics
Self-healing ceramics are a class of materials that can repair cracks or damage through various mechanisms, such as oxidation, precipitation, or phase transformation. Some examples of self-healing ceramics include:
-
Oxidation-based self-healing ceramics: Ceramics that can form a protective oxide layer at the crack surface, filling the crack and preventing its propagation.
-
Precipitation-based self-healing ceramics: Ceramics that can form precipitates, such as silicates or aluminates, at the crack surface, filling the crack and restoring the material's properties.
-
Phase transformation-based self-healing ceramics: Ceramics that can undergo a phase transformation, such as from tetragonal to monoclinic, at the crack tip, inducing a compressive stress that closes the crack.
Composites
Self-healing composites are materials that combine two or more distinct phases, such as a polymer matrix and a reinforcing fiber, with a self-healing capability. The self-healing mechanism can be incorporated into the matrix, the reinforcement, or the interface between them. Some examples of self-healing composites include:
-
Capsule-based self-healing composites: Composites containing microcapsules filled with a healing agent that is released upon damage, similar to capsule-based self-healing polymers.
-
Vascular self-healing composites: Composites containing a network of channels or fibers that deliver the healing agent to the damage site, similar to vascular self-healing polymers.
-
Intrinsic self-healing composites: Composites containing a matrix or reinforcement with intrinsic self-healing properties, such as a supramolecular polymer or a shape memory alloy.
Applications of Self-Healing Materials
Self-healing materials have a wide range of potential applications across various industries, from aerospace and automotive to construction and electronics. Some of the most promising applications of self-healing materials include:
Aerospace
In the aerospace industry, self-healing materials can be used to increase the durability and reliability of aircraft components, such as wings, fuselages, and engines. For example:
-
Self-healing composites can be used to repair damage caused by impact, fatigue, or environmental factors, such as moisture or temperature variations.
-
Self-healing coatings can be used to protect aircraft surfaces from corrosion, erosion, or UV radiation, extending their lifespan and reducing maintenance costs.
Automotive
In the automotive industry, self-healing materials can be used to enhance the safety, performance, and sustainability of vehicles. For example:
-
Self-healing polymers can be used to create lightweight and resilient components, such as bumpers, panels, or tires, that can recover from minor impacts or punctures.
-
Self-healing metals can be used to create high-strength and fatigue-resistant parts, such as engine components or suspension systems, that can withstand repeated stress and strain.
Construction
In the construction industry, self-healing materials can be used to improve the durability and sustainability of buildings and infrastructure. For example:
-
Self-healing concrete can be used to repair cracks and prevent moisture ingress, extending the lifespan of structures and reducing maintenance costs.
-
Self-healing asphalt can be used to create more resilient and sustainable roads that can recover from damage caused by traffic, weather, or aging.
Electronics
In the electronics industry, self-healing materials can be used to create more reliable and adaptable devices, such as sensors, displays, or batteries. For example:
-
Self-healing polymers can be used to create flexible and stretchable electronics that can recover from mechanical damage, such as bending or twisting.
-
Self-healing conductors can be used to create self-repairing circuits or interconnects that can maintain their electrical properties after damage.
Biomedicine
In the biomedical field, self-healing materials can be used to create more biocompatible and functional implants, scaffolds, or drug delivery systems. For example:
-
Self-healing hydrogels can be used to create injectable or implantable materials that can adapt to the body's dynamic environment and promote tissue regeneration.
-
Self-healing polymers can be used to create self-sealing wound dressings or sutures that can prevent infection and promote healing.
These are just a few examples of the potential applications of self-healing materials, and the list is likely to grow as the field continues to evolve and mature.
Challenges and Future Directions
Despite the significant progress made in the development of self-healing materials, there are still several challenges and opportunities for future research. Some of the key challenges and future directions for self-healing materials include:
Scaling up and Manufacturing
One of the main challenges in the commercialization of self-healing materials is the scaling up and manufacturing of these materials in a cost-effective and reliable way. Many self-healing materials require complex and precise fabrication methods, such as microencapsulation or 3D printing, that can be difficult to scale up to industrial levels. Additionally, the incorporation of self-healing components, such as microcapsules or vascular networks, can affect the processing and properties of the base material, requiring careful optimization and quality control.
Future research in this area should focus on developing scalable and efficient manufacturing methods for self-healing materials, such as roll-to-roll processing, extrusion, or injection molding. This may require the development of new materials, such as self-healing thermoplastics or elastomers, that are more compatible with existing manufacturing processes. Additionally, the development of in-line monitoring and control systems can help ensure the consistency and reliability of self-healing materials during production.
Improving Healing Efficiency and Repeatability
Another challenge in the development of self-healing materials is improving their healing efficiency and repeatability, particularly for applications that require multiple healing cycles or complete recovery of properties. Many self-healing materials have limited healing efficiency, typically less than 100%, due to factors such as incomplete polymerization, poor interfacial bonding, or residual damage. Additionally, the healing efficiency and repeatability can degrade over time due to factors such as depletion of healing agents, accumulation of damage, or changes in the material's microstructure.
Future research in this area should focus on developing new healing mechanisms and materials that can provide higher healing efficiency and repeatability, such as multi-stage healing systems, adaptive healing agents, or self-replenishing vascular networks. Additionally, the development of non-destructive testing and monitoring methods can help assess the healing performance and remaining useful life of self-healing materials in real-time.
Enhancing Multifunctionality and Responsiveness
Another opportunity for future research in self-healing materials is enhancing their multifunctionality and responsiveness, i.e., their ability to perform multiple functions or adapt to changing environmental conditions. Many self-healing materials are designed to repair a specific type of damage, such as cracks or scratches, but they may not be able to address other types of degradation, such as corrosion, wear, or aging. Additionally, self-healing materials may not be able to respond to changes in their operating conditions, such as temperature, humidity, or mechanical stress, which can affect their performance and durability.
Future research in this area should focus on developing self-healing materials that can address multiple types of damage or degradation, such as self-healing anticorrosion coatings or self-lubricating composites. Additionally, the development of stimuli-responsive self-healing materials that can adapt their healing behavior based on external stimuli, such as light, heat, or pH, can enable more dynamic and efficient healing. The integration of self-healing materials with other functional materials, such as sensors, actuators, or energy harvesters, can also create new opportunities for multifunctional and responsive systems.
Understanding and Modeling Self-Healing Mechanisms
A key challenge in the rational design and optimization of self-healing materials is understanding and modeling the complex physical, chemical, and biological mechanisms involved in the healing process. Self-healing in materials can involve a wide range of phenomena, such as mass transport, phase transformation, chemical reaction, and mechanical deformation, which can occur across multiple length and time scales. Additionally, the healing performance can be influenced by various factors, such as the material composition, microstructure, damage type, and environmental conditions.
Future research in this area should focus on developing advanced characterization and modeling tools that can probe the self-healing mechanisms at different scales, such as in-situ microscopy, spectroscopy, or tomography. The development of multiscale and multiphysics computational models that can predict the healing behavior and properties of self-healing materials based on their composition and microstructure can also guide the design and optimization of these materials. The integration of machine learning and data-driven approaches with physics-based models can also accelerate the discovery and development of new self-healing materials.
Conclusion
Self-healing materials are a rapidly growing and promising field that has the potential to revolutionize various industries, from aerospace and automotive to construction and electronics. By enabling materials to repair themselves autonomously or with minimal external intervention, self-healing materials can increase the durability, reliability, and sustainability of products and structures, while reducing maintenance costs and extending their lifespan.
The development of self-healing materials has made significant progress in recent years, with the demonstration of various healing mechanisms and materials, such as capsule-based polymers, vascular composites, and intrinsic ceramics. These materials have shown impressive healing efficiencies and repeatability, with some being able to recover up to 100% of their original properties after multiple damage-healing cycles.
However, there are still several challenges and opportunities for future research in self-healing materials, such as scaling up and manufacturing, improving healing efficiency and repeatability, enhancing multifunctionality and responsiveness, and understanding and modeling self-healing mechanisms. Addressing these challenges will require a multidisciplinary and collaborative approach, involving experts from materials science, chemistry, physics, biology, and engineering.
As the field of self-healing materials continues to evolve and mature, we can expect to see more innovative and transformative applications emerge, from self-healing spacecraft and vehicles to self-repairing buildings and electronics. The development of self-healing materials can also have significant implications for sustainability and circular economy, by reducing waste and extending the useful life of products and materials.
In conclusion, self-healing materials represent a paradigm shift in the way we design and use materials, from passive and disposable to active and adaptable. By harnessing the power of self-healing, we can create a more resilient, sustainable, and intelligent world, where materials can heal themselves and adapt to changing conditions, just like living organisms. The future of self-healing materials is bright and full of exciting possibilities, and we can look forward to seeing more breakthroughs and innovations in this field in the years to come.