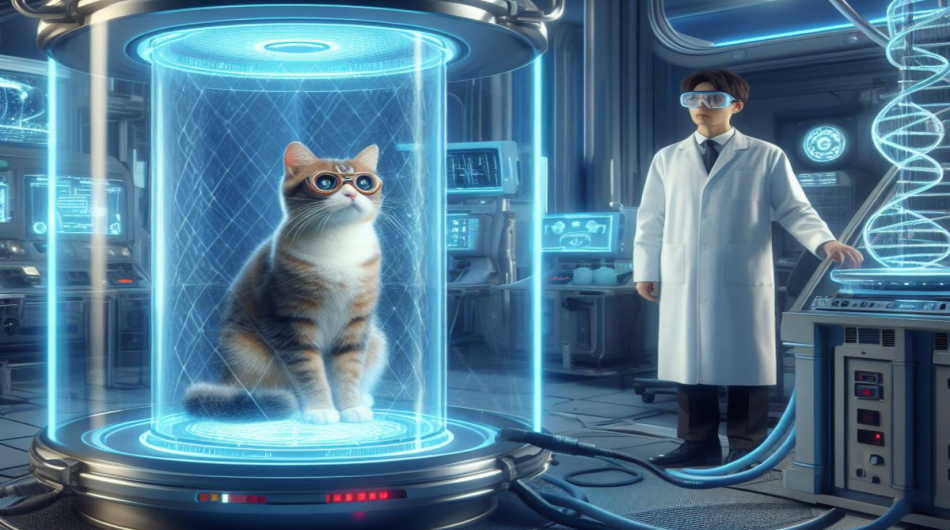
Quantum teleportation is a remarkable phenomenon in quantum physics that allows the transfer of quantum information from one location to another without the need for the physical transmission of the quantum system itself. This process is not to be confused with the science fiction concept of teleportation, where matter is instantly transported from one place to another. Instead, quantum teleportation involves the transfer of the quantum state of a particle, such as its spin or polarization, to another particle that may be located far away. This is achieved through the use of quantum entanglement, a peculiar property of quantum systems that allows them to be connected in ways that are not possible in classical physics. Quantum teleportation has significant implications for the development of quantum communication and quantum computing, and has been experimentally demonstrated in various physical systems, from photons to atoms and even to superconducting circuits.
The Basics of Quantum Teleportation
Quantum States and Qubits
To understand quantum teleportation, it is essential to first grasp the concept of quantum states and qubits. In quantum mechanics, a quantum state is a mathematical description of a quantum system, such as an atom, electron, or photon. Unlike classical systems, which can be described by definite values of physical quantities like position and momentum, quantum systems are characterized by a wave function that encodes the probabilities of finding the system in different states when measured.
The basic unit of quantum information is the quantum bit, or qubit. While a classical bit can only be in one of two states (0 or 1), a qubit can be in a superposition of both states simultaneously. This means that a qubit can be in a linear combination of the states |0⟩ and |1⟩, which are known as the computational basis states. The general state of a qubit can be written as:
|ψ⟩ = α|0⟩ + β|1⟩
where α and β are complex numbers satisfying |α|² + |β|² = 1. The values of α and β determine the probabilities of measuring the qubit in the |0⟩ or |1⟩ state, respectively.
Quantum Entanglement
Quantum entanglement is a key concept in quantum teleportation and quantum information in general. Entanglement occurs when two or more quantum systems are correlated in such a way that the state of one system cannot be described independently of the others, even when the systems are separated by large distances. This is in stark contrast to classical correlations, which are limited by the speed of light and decrease with distance.
A simple example of an entangled state is the Bell state, also known as an EPR pair, named after the physicists Einstein, Podolsky, and Rosen. The Bell state is a two-qubit state that can be written as:
|Φ⁺⟩ = (|00⟩ + |11⟩) / √2
This state represents a superposition of the two qubits being either both in the |0⟩ state or both in the |1⟩ state. If one qubit is measured in the computational basis, the other qubit will always be found in the same state, regardless of the distance between them. This is the essence of entanglement: the states of the two qubits are correlated in a way that cannot be explained by classical physics.
The Quantum Teleportation Protocol
The quantum teleportation protocol, proposed by Charles Bennett, Gilles Brassard, Claude Crépeau, Richard Jozsa, Asher Peres, and William Wootters in 1993, is a procedure that allows the transfer of an unknown quantum state from one location to another using entanglement and classical communication. The protocol involves three parties: Alice, who possesses the quantum state to be teleported; Bob, who receives the teleported state; and an entangled pair of qubits shared between Alice and Bob.
The steps of the quantum teleportation protocol are as follows:
-
Alice and Bob share a pair of entangled qubits, such as a Bell state, with one qubit held by each party.
-
Alice has a third qubit in an unknown state |ψ⟩ that she wants to teleport to Bob.
-
Alice performs a joint measurement on her two qubits (the unknown state and her half of the entangled pair) in the Bell basis. This measurement entangles the unknown state with her half of the entangled pair and collapses the entanglement between Alice and Bob.
-
Alice sends the classical results of her measurement (two bits of information) to Bob via a classical communication channel.
-
Based on the classical information received from Alice, Bob performs a specific quantum operation on his half of the entangled pair to reconstruct the original state |ψ⟩.
It is important to note that the quantum teleportation protocol does not violate the laws of quantum mechanics or relativity. The unknown quantum state is not transmitted faster than the speed of light, as classical communication is required to complete the teleportation process. Moreover, the original state is destroyed during Alice's measurement, ensuring that the no-cloning theorem of quantum mechanics is not violated.
Experimental Realizations of Quantum Teleportation
Since the proposal of the quantum teleportation protocol, numerous experimental demonstrations have been carried out in various physical systems. These experiments have not only confirmed the feasibility of quantum teleportation but have also pushed the limits of the distance over which quantum states can be teleported and the fidelity of the teleported states.
Photonic Quantum Teleportation
Photons, the quantum particles of light, have been the workhorse of quantum teleportation experiments due to their low interaction with the environment and the ease with which they can be manipulated and transmitted over long distances. The first experimental demonstration of quantum teleportation was achieved in 1997 by Anton Zeilinger's group at the University of Innsbruck, Austria, using polarization-entangled photon pairs.
Since then, photonic quantum teleportation has been realized over increasingly longer distances, both in optical fibers and in free space. In 2012, a team led by Anton Zeilinger achieved quantum teleportation over a distance of 143 km between the Canary Islands of La Palma and Tenerife, setting a new record for the distance over which quantum states have been teleported. This experiment used entangled photon pairs generated by a laser source on La Palma and detected by receivers on both islands.
More recently, in 2017, a Chinese team led by Jian-Wei Pan achieved satellite-based quantum teleportation, demonstrating the transfer of quantum states between a ground station and the Micius satellite, which was in a low Earth orbit at an altitude of around 500 km. This experiment opened up new possibilities for global-scale quantum communication and the development of quantum networks.
Atomic and Ionic Quantum Teleportation
While photons are ideal for transmitting quantum information over long distances, atoms and ions are promising candidates for processing and storing quantum information in quantum computers and memories. Quantum teleportation has also been demonstrated in these systems, albeit over shorter distances compared to photonic experiments.
In 2004, a team led by David Wineland at the National Institute of Standards and Technology (NIST) in Boulder, Colorado, demonstrated quantum teleportation between two trapped ionic qubits separated by a few micrometers. The qubits were encoded in the internal electronic states of beryllium ions, and the entanglement was generated through a series of laser pulses that coupled the ions' internal states with their collective motion.
Quantum teleportation has also been achieved in neutral atomic systems, such as rubidium atoms in optical lattices or dipole traps. In 2006, a team led by Immanuel Bloch at the Max Planck Institute of Quantum Optics in Garching, Germany, demonstrated quantum teleportation between two atomic qubits separated by a few micrometers in an optical lattice. The entanglement was generated through a process called collisional entanglement, where the atoms' internal states become correlated through controlled collisions.
Superconducting Circuit Quantum Teleportation
Superconducting circuits, which consist of Josephson junctions and microwave resonators, are another promising platform for quantum computing and quantum information processing. These circuits operate at cryogenic temperatures and can be engineered to have long coherence times and strong interactions between qubits.
In 2014, a team led by Andreas Wallraff at ETH Zurich, Switzerland, demonstrated deterministic quantum teleportation between two superconducting qubits on a single microchip. The qubits were encoded in the electronic states of transmon qubits, which are a type of superconducting qubit that is insensitive to charge noise. The entanglement was generated through a process called quantum bus coupling, where the qubits interact through a shared microwave resonator.
More recently, in 2019, a team led by Jian-Wei Pan and Haohua Wang at the University of Science and Technology of China achieved quantum teleportation between two superconducting qubits separated by a few millimeters on a multi-chip device. This experiment demonstrated the feasibility of chip-to-chip quantum teleportation, which is a key step towards building larger-scale quantum computing systems.
Applications of Quantum Teleportation
Quantum teleportation is not only a fascinating phenomenon in its own right but also has important practical applications in quantum communication, quantum computing, and quantum cryptography.
Quantum Communication and Networks
One of the main applications of quantum teleportation is in the development of quantum communication systems and networks. By using entanglement and quantum teleportation, it is possible to transmit quantum information securely over long distances, without the risk of eavesdropping or interception.
Quantum communication protocols, such as quantum key distribution (QKD), rely on the distribution of entangled photon pairs between remote parties to establish a shared secret key that can be used for secure communication. Quantum teleportation can be used to extend the range of QKD systems by allowing the transfer of quantum states between intermediate nodes in a quantum network.
Moreover, quantum teleportation can enable the realization of quantum repeaters, which are devices that can overcome the limitations of photon loss and decoherence in long-distance quantum communication. Quantum repeaters work by distributing entanglement over shorter distances, performing entanglement swapping and purification operations to generate long-distance entangled pairs, and using quantum teleportation to transfer quantum states between the nodes.
Quantum Computing and Error Correction
Quantum teleportation also plays a crucial role in quantum computing, particularly in the implementation of quantum error correction codes and fault-tolerant quantum computation. Quantum computers are prone to errors due to the fragility of quantum states and the effects of environmental noise and decoherence. Quantum error correction codes are designed to detect and correct these errors by encoding logical qubits into larger entangled states and performing syndrome measurements and correction operations.
Quantum teleportation is a key ingredient in many quantum error correction codes, such as the surface code and the color code. In these codes, logical qubits are encoded into a lattice of physical qubits, and quantum teleportation is used to perform the syndrome measurements and correction operations without disturbing the encoded information. By teleporting the error syndromes to ancillary qubits and measuring them, it is possible to detect and correct errors without directly measuring the logical qubits.
Furthermore, quantum teleportation can be used to implement fault-tolerant quantum gates, which are quantum operations that can be performed reliably even in the presence of errors. By teleporting quantum states through a series of error-corrected gates, it is possible to achieve reliable quantum computation with imperfect hardware.
Quantum Cryptography and Blind Quantum Computing
Quantum teleportation also has applications in quantum cryptography and secure quantum computing. In particular, quantum teleportation is a key component of protocols for blind quantum computation, where a client can delegate a quantum computation to a remote server without revealing the input data or the details of the computation.
In a typical blind quantum computing protocol, the client prepares a set of single-qubit states that encode the input data and the desired computation, and sends them to the server. The server entangles these qubits with its own ancillary qubits and performs a series of quantum gates and measurements according to the client's instructions. The server then sends the measurement results back to the client, who can use them to reconstruct the output of the computation.
By using quantum teleportation, the client can send the input qubits to the server in a way that preserves their privacy and security. The client can prepare the input qubits in random states and teleport them to the server using pre-shared entangled pairs. The server can then perform the computation on the teleported qubits without learning anything about their original states. At the end of the computation, the server teleports the output qubits back to the client, who can decrypt them using the classical information from the teleportation protocol.
Blind quantum computing has important applications in secure cloud computing and delegated quantum computing scenarios, where users may want to perform quantum computations on untrusted or remote hardware without compromising the privacy and security of their data.
Challenges and Future Directions
Despite the significant progress made in the experimental realization and applications of quantum teleportation, there are still many challenges and open questions that need to be addressed to fully harness the potential of this technology.
Scaling Up Quantum Teleportation
One of the main challenges in quantum teleportation is scaling up the systems to larger numbers of qubits and longer distances. While quantum teleportation has been demonstrated in various physical systems, the fidelity and success rates of these experiments are still limited by the quality of the entanglement generation, the efficiency of the quantum operations, and the effects of noise and decoherence.
To achieve practical quantum communication and computation, it will be necessary to develop more robust and scalable methods for generating and distributing entanglement, performing quantum gates and measurements, and error correction. This will require advances in materials science, nanofabrication, and quantum control techniques, as well as the development of new protocols and architectures for quantum teleportation and error correction.
Integrating Quantum Teleportation with Classical Networks
Another challenge is the integration of quantum teleportation with classical communication networks and infrastructure. Quantum communication systems rely on the transmission of both quantum and classical information, and the efficient coordination and synchronization of these two types of information is essential for the reliable operation of quantum networks.
Moreover, quantum communication systems will need to be compatible with existing classical networks and protocols, such as the internet and telecommunication networks, to enable the seamless integration of quantum and classical information processing. This will require the development of quantum-classical interfaces, such as quantum modems and routers, as well as the standardization of quantum communication protocols and architectures.
Exploring New Physical Platforms and Encodings
While quantum teleportation has been demonstrated in various physical systems, there is still much room for exploration and innovation in terms of new platforms and encodings for quantum information. For example, hybrid quantum systems that combine the advantages of different physical platforms, such as the long coherence times of atoms and the strong interactions of superconducting circuits, could enable more efficient and scalable quantum teleportation and computation.
Moreover, new types of quantum encodings, such as continuous-variable quantum information and topological quantum codes, could offer new possibilities for quantum teleportation and error correction. Continuous-variable quantum information, which is based on the quadratures of electromagnetic fields, could enable the teleportation of quantum states with higher dimensionality and information capacity. Topological quantum codes, which encode quantum information in the global properties of many-body systems, could provide intrinsic robustness against local errors and decoherence.
Developing Quantum Teleportation Applications and Protocols
Finally, there is a need for the development of new applications and protocols for quantum teleportation, beyond the existing ones in quantum communication and computation. Quantum teleportation could have potential applications in other areas of quantum information science, such as quantum metrology, quantum sensing, and quantum simulation.
For example, quantum teleportation could be used to enhance the sensitivity and resolution of quantum sensors and imaging systems, by allowing the transfer of delicate quantum states between remote sensors and detectors. Quantum teleportation could also be used to distribute entanglement and correlations in quantum simulators, enabling the study of complex many-body systems and the simulation of exotic quantum phenomena.
Moreover, new protocols for quantum teleportation, such as port-based teleportation and probabilistic teleportation, could offer advantages in terms of resource efficiency and success probability compared to the standard teleportation protocol. Port-based teleportation, which uses a large entangled state as a resource for teleportation, could enable the deterministic teleportation of quantum states without the need for classical communication. Probabilistic teleportation, which relies on post-selection and heralding, could enable the teleportation of quantum states with higher fidelity and success rates, at the cost of a lower overall throughput.
Conclusion
Quantum teleportation is a remarkable phenomenon that showcases the power and peculiarities of quantum mechanics. By harnessing the properties of entanglement and superposition, quantum teleportation allows the transfer of quantum information across space without the physical transmission of the quantum system itself. This has important implications for the development of quantum communication, quantum computing, and quantum cryptography, as well as for our understanding of the nature of quantum information and its relationship to space and time.
Since its theoretical proposal in 1993, quantum teleportation has been experimentally demonstrated in a variety of physical systems, from photons to atoms and superconducting circuits, and over increasingly longer distances and with higher fidelities.