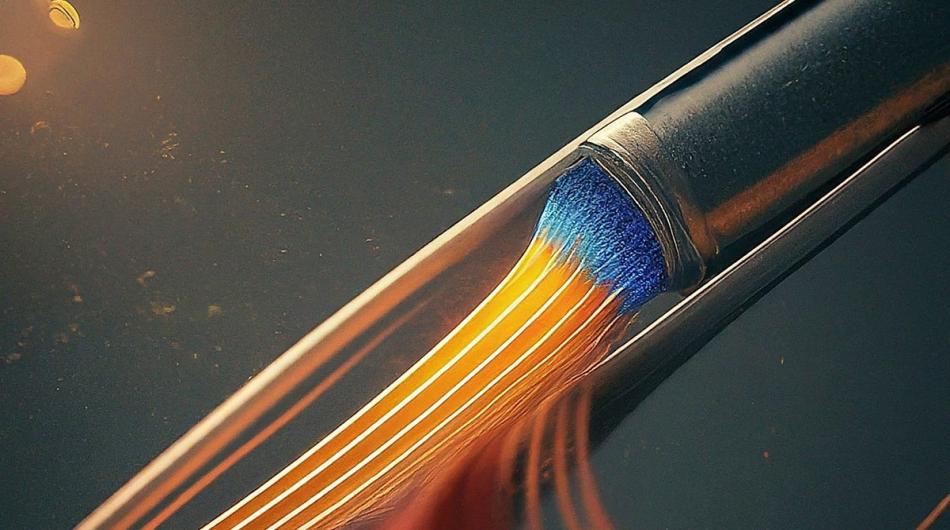
Photonic Integrated Circuits (PICs) represent a revolutionary advancement in the field of integrated photonics, where multiple photonic functions are combined on a single semiconductor substrate. Unlike electronic integrated circuits (ICs) that use electrons to perform operations, PICs use photons (light particles) for processing, manipulating, and transmitting information. This integration of photonic components onto a single chip has paved the way for the development of sophisticated and compact optical systems with numerous applications.
Historical Context and Evolution of PICs
The journey of PICs began in the 1960s with the advent of the laser and the rapid advancements in fiber optics technology. The early photonic circuits, often referred to as photonic integrated chips, were relatively simple and combined only a few optical components. Over the decades, driven by continuous progress in materials science, fabrication techniques, and design methodologies, PICs have evolved significantly.
The transition from traditional photonics, which involved discrete optical components, to integrated optical circuits has marked a significant milestone in the field. This shift towards integration allows for the miniaturization of optical systems, leading to enhanced performance, reduced cost, and increased reliability. The modern photonic IC incorporates a variety of components such as waveguides, modulators, detectors, and lasers, all integrated on a single chip to perform complex optical functions.
Key Terminology and Basic Principles of PICs
To understand the concept of photonic integrated circuits, it is essential to familiarize oneself with some critical terminology and basic principles:
Integrated Photonics Integrated photonics refers to the technology of integrating multiple photonic devices and circuits on a common platform. This technology aims to combine various optical functions into a compact and efficient system, using materials like silicon, indium phosphide, or silicon nitride.
Photonic Integrated Circuit (PIC) A PIC is a circuit that integrates several photonic components and functions onto a single semiconductor chip. These circuits manipulate light to perform tasks such as signal processing, modulation, and switching, which are essential in high-speed communication systems.
Integrated Optical Circuit An integrated optical circuit is another term for a PIC. It emphasizes the integration of various optical components, such as waveguides, modulators, detectors, and lasers, into a single, miniaturized device.
Waveguide A waveguide is a structure that guides light waves along a specific path within a PIC. Waveguides are typically made from materials like silicon or silicon nitride and are essential for directing light between different components on the chip.
Modulator A modulator is a device used in PICs to control and modify the properties of light, such as its intensity, phase, or wavelength. Modulators are crucial for encoding information onto light signals in communication systems.
Detector A detector is a component in a PIC that converts light signals into electrical signals. Detectors are vital for receiving and interpreting optical data in various applications, including communication and sensing.
Multiplexer/Demultiplexer A multiplexer combines multiple optical signals into a single signal for transmission over a shared medium, while a demultiplexer separates a combined signal back into individual signals. These components are essential for efficient use of bandwidth in optical networks.
By integrating these components and more, PICs enable the creation of complex optical systems that are smaller, faster, and more energy-efficient than traditional optical technologies. This integration not only enhances the performance of optical systems but also opens up new possibilities in telecommunications, data centers, computing, and beyond
Design and Fabrication of PICs
Design of Photonic Integrated Circuits (PICs)
Design Aspects of Photonic ICs
Designing photonic integrated circuits (PICs) involves several critical steps that ensure the integrated photonic devices function efficiently. The design process typically starts with defining the required optical functions, such as modulation, switching, filtering, or amplification. These functions determine the types of photonic components to be integrated onto the chip.
- Component Design: Each photonic component, such as waveguides, modulators, detectors, and lasers, must be carefully designed. The properties of these components, including their size, shape, and refractive index, are tailored to control the propagation of light effectively. For instance, waveguides are designed to confine and guide light with minimal loss.
- Circuit Layout: Once individual components are designed, they are arranged in a layout that optimizes the overall performance of the PIC. This involves placing components in a way that minimizes optical losses, reduces crosstalk, and ensures efficient coupling between different elements. Advanced computer-aided design (CAD) tools are used to simulate and refine the layout.
- Material Selection: The choice of material platform is crucial in PIC design. Common materials include silicon, indium phosphide (InP), and silicon nitride. Silicon is widely used due to its compatibility with existing semiconductor fabrication processes and its excellent optical properties for guiding light. InP is preferred for applications requiring active components like lasers and modulators because of its direct bandgap.
- Integration Strategy: Depending on the application, designers may opt for monolithic integration (all components on a single chip) or hybrid integration (combining different material platforms). Hybrid integration allows for the combination of the best properties of different materials, such as the low-loss waveguiding of silicon and the efficient light emission of InP.
Fabrication of Photonic ICs
Fabrication Processes and Techniques
Fabricating PICs involves a series of sophisticated processes that translate the design into a functional integrated circuit. The main fabrication steps include:
- Photolithography: This process involves transferring the circuit layout onto a substrate using light. A light-sensitive material (photoresist) is coated onto the substrate, exposed to a pattern of light, and then developed to create a mask for subsequent etching or deposition steps.
- Etching: Etching removes material from the substrate to create the designed photonic structures. There are two main types of etching: dry etching (using plasma) and wet etching (using chemical solutions). Dry etching is preferred for its precision and ability to create high-aspect-ratio structures.
- Deposition: Material deposition techniques, such as chemical vapor deposition (CVD) or physical vapor deposition (PVD), are used to add thin films of materials onto the substrate. These films form the active and passive layers of the PIC.
- Doping: Doping involves introducing impurities into the semiconductor material to modify its electrical and optical properties. This step is crucial for creating active components like lasers and modulators.
- Planarization: Chemical mechanical polishing (CMP) is used to smooth the surface of the wafer, ensuring that subsequent layers are deposited on a flat surface. This step is important for maintaining the integrity of the optical paths within the PIC.
- Metallization: Metal layers are deposited to form electrical contacts and interconnects. This step ensures that the photonic components can be controlled and powered by electrical signals.
Material Platforms
Different material platforms offer unique advantages for PICs:
- Silicon: Ideal for passive components like waveguides due to its low optical loss and compatibility with CMOS technology. Silicon photonics leverages the mature semiconductor industry infrastructure.
- Indium Phosphide (InP): Suitable for active components like lasers and modulators due to its direct bandgap properties, enabling efficient light emission.
- Silicon Nitride (SiN): Offers low loss at a broad range of wavelengths, making it ideal for applications requiring precise wavelength control, such as filters and resonators.
Packaging and Testing of PICs
Packaging Challenges
Packaging PICs involves enclosing the delicate photonic components in a protective shell while ensuring efficient optical and electrical connections. Key challenges include:
- Thermal Management: Efficient heat dissipation is crucial to maintain the performance and reliability of PICs, especially for components that generate heat, such as lasers.
- Optical Alignment: Precise alignment of optical fibers or waveguides with the photonic components is essential to minimize coupling losses.
- Environmental Protection: Packaging must protect the PIC from environmental factors like moisture, dust, and mechanical stress.
Testing Methodologies
Ensuring the functional integrity of PICs requires rigorous testing at various stages of fabrication and post-fabrication:
- On-Wafer Testing: Early testing is performed directly on the wafer before it is diced into individual chips. This includes optical characterization of waveguides, modulators, and other components to ensure they meet design specifications.
- Die-Level Testing: After dicing, individual dies are tested to verify their performance. This involves measuring parameters such as insertion loss, return loss, modulation efficiency, and detector sensitivity.
- System-Level Testing: Once packaged, the PIC is tested as part of an integrated optical circuit. This step ensures that the PIC functions correctly within the larger system, such as a telecommunications network or data center.
- Reliability Testing: Long-term reliability tests, including thermal cycling, humidity exposure, and mechanical stress tests, are conducted to ensure the PIC's durability and performance over time.
By addressing these design, fabrication, packaging, and testing aspects, photonic ICs can achieve high performance, reliability, and efficiency, enabling their widespread adoption in modern technology applications.
Applications of Photonic Integrated Circuits
Telecommunications and Data Centers
Telecommunications
Photonic Integrated Circuits (PICs) are pivotal in advancing telecommunications technology. These circuits, often referred to as photonic integrated chips, integrate various optical components like lasers, modulators, and detectors onto a single chip, enabling efficient and high-speed data transmission.
- Dense Wavelength Division Multiplexing (DWDM): PICs facilitate DWDM by allowing multiple wavelengths (channels) of light to be transmitted simultaneously through a single optical fiber. This significantly increases the data capacity of telecommunications networks.
- Optical Transceivers: PICs are used in optical transceivers, which convert electrical signals to optical signals and vice versa. These transceivers are essential for high-speed data communication over long distances with minimal signal loss.
- Switches and Routers: PICs enable the development of optical switches and routers, which can manage and direct large volumes of data traffic efficiently, reducing latency and power consumption compared to electronic counterparts.
Data Centers
In data centers, the demand for high bandwidth, low latency, and energy efficiency is paramount. PICs play a crucial role in meeting these demands:
- Optical Interconnects: PICs are used to create optical interconnects that link different servers, storage systems, and networking equipment within data centers. These interconnects offer higher data transfer rates and lower power consumption compared to traditional copper-based connections.
- Data Processing: PICs enhance the speed and efficiency of data processing by enabling faster communication between processors and memory modules. This is particularly important for applications requiring real-time data analysis and high-performance computing.
Sensing and Imaging
Medical Technologies
PICs are revolutionizing medical sensing and imaging technologies, offering high sensitivity, compactness, and the ability to integrate multiple sensing functions on a single chip:
- Biosensors: PIC-based biosensors are used for detecting specific biological molecules, such as proteins, DNA, or pathogens. These sensors offer high sensitivity and specificity, making them valuable for medical diagnostics and monitoring.
- Optical Coherence Tomography (OCT): PICs are employed in OCT systems, which are used for high-resolution imaging of biological tissues. This technology is widely used in ophthalmology for retinal imaging and in other medical fields for imaging internal tissues.
Environmental Monitoring
PICs are also making significant impacts in environmental sensing and monitoring:
- Air and Water Quality Sensors: PICs can be used to develop compact and sensitive sensors for monitoring air and water quality. These sensors can detect pollutants and hazardous substances at very low concentrations, providing valuable data for environmental protection.
- Remote Sensing: PICs enable the development of lightweight and efficient remote sensing devices used in environmental monitoring, agriculture, and meteorology. These devices can be deployed in drones or satellites to gather data over large areas.
Emerging Fields: Photonic Computing and Quantum Technologies
Photonic Computing
Photonic computing is an emerging field where PICs are poised to transform how data is processed and computed:
- Optical Processors: PICs are used to create optical processors that perform computations using light instead of electricity. This approach can potentially offer much higher processing speeds and lower power consumption than traditional electronic processors.
- Neuromorphic Computing: PICs are being explored for neuromorphic computing systems, which mimic the neural architecture of the human brain. These systems aim to perform complex cognitive tasks with greater efficiency and speed.
Quantum Technologies
PICs are at the forefront of quantum technologies, enabling advances in quantum computing and quantum communication:
- Quantum Computing: PICs can be used to develop quantum circuits that manipulate quantum bits (qubits) using light. These circuits are essential for building scalable and reliable quantum computers that can solve problems beyond the reach of classical computers.
- Quantum Communication: PICs facilitate the development of secure quantum communication systems. By leveraging the principles of quantum mechanics, these systems can provide unbreakable encryption and secure data transmission.
Market Trends and Future Prospects
Current Market Scenario
Major Players
The photonic integrated circuit (PIC) market is witnessing significant growth, driven by the increasing demand for high-speed data transmission and the advancement of integrated photonics. Several key players dominate this market, including:
- Intel Corporation: Known for its silicon photonics technology, Intel has made substantial advancements in integrating photonic components with its semiconductor products, particularly for data centers and telecommunications.
- Cisco Systems: Cisco has been active in developing PIC-based solutions for networking and data communications, emphasizing high-speed and energy-efficient optical interconnects.
- Infinera Corporation: A leader in optical networking, Infinera focuses on developing photonic ICs for high-capacity optical transport networks, leveraging its expertise in indium phosphide (InP) technology.
- NeoPhotonics Corporation: Specializing in advanced photonic components, NeoPhotonics designs and manufactures PICs for high-speed communications, including coherent optics and 400G solutions.
- Lumentum Holdings Inc.: Lumentum provides PIC-based products for telecommunications and data center applications, including optical transceivers and components for dense wavelength division multiplexing (DWDM).
Recent Product Launches
Recent product launches highlight the advancements in integrated photonics and photonic IC technologies:
- Intel's 400G Optical Transceivers: Intel launched 400G optical transceivers leveraging silicon photonics to meet the growing demand for high-speed data transfer in data centers.
- Infinera's XR Optics: Infinera introduced XR Optics, a coherent optical subsystem using PIC technology designed to significantly reduce network costs and complexity.
- Cisco's Silicon One: Cisco's Silicon One platform integrates photonic ICs for high-performance networking, aiming to deliver unprecedented bandwidth and efficiency.
Future Trends and Innovative Research
Potential Advancements and Breakthroughs
The future of PICs is marked by several promising trends and research directions:
- Higher Integration Levels: Ongoing research aims to integrate more optical components and functions onto a single photonic IC, enhancing performance and reducing costs. This includes the development of fully integrated optical transceivers and on-chip lasers.
- Silicon Photonics: Advances in silicon photonics are expected to continue, with efforts focused on improving the performance and scalability of silicon-based PICs. Innovations such as heterogeneous integration, combining silicon with other materials like InP, are gaining traction.
- Quantum Photonics: PICs are crucial for developing scalable quantum computing and communication systems. Research is directed toward integrating quantum light sources, modulators, and detectors on a single chip to build practical quantum photonic circuits.
- 3D Photonic Integration: Researchers are exploring three-dimensional photonic integration to pack more functionality into a smaller footprint, enabling more complex and efficient photonic systems.
Technical and Market Challenges
Technical Challenges
- Thermal Management: Managing heat dissipation in PICs is crucial, especially as integration levels increase. Effective thermal management solutions are needed to maintain performance and reliability.
- Fabrication Precision: Achieving high precision in the fabrication of photonic components is challenging. Variations in manufacturing can lead to performance discrepancies, requiring stringent process controls.
- Material Limitations: The choice of materials impacts the efficiency and functionality of PICs. While silicon is prevalent, its indirect bandgap limits certain applications, necessitating the integration of other materials like InP.
Market Challenges
- Cost: The initial cost of developing and producing PICs can be high, which may slow adoption in cost-sensitive markets. However, economies of scale and advancements in manufacturing processes are expected to reduce costs over time.
- Standardization: The lack of standardization in PIC design and manufacturing poses a challenge. Developing industry standards can facilitate interoperability and wider adoption of PIC technologies.
- Competition with Electronic Solutions: Despite their advantages, PICs compete with advanced electronic ICs. Overcoming market inertia and demonstrating clear benefits is essential for broader market penetration.
Opportunities for Growth and Development
- Expanding Applications: As PIC technology matures, new applications in fields such as autonomous vehicles, healthcare, and environmental monitoring are emerging, providing substantial growth opportunities.
- Collaborative Research: Increased collaboration between academia, industry, and government can accelerate innovation in PIC technology. Public-private partnerships and funding initiatives can drive research and development efforts.
-
Emerging Markets: Regions like Asia-Pacific are witnessing rapid growth in telecommunications and data centers, presenting lucrative markets for PIC-based solutions.
Conclusion and Future Outlook
Summary of Critical Points
- Throughout this article, we have explored the fundamental aspects and significance of Photonic Integrated Circuits (PICs) across various sections:
- Introduction to PICs: We introduced PICs, emphasizing their role in integrating multiple photonic functions on a single chip, and discussed their evolution from traditional photonics to integrated photonics, highlighting the advantages over electronic integrated circuits.
- Design and Fabrication of PICs: The design process of photonic ICs involves careful planning of optical components and circuit layout, selection of suitable materials, and strategic integration. The fabrication techniques, including photolithography, etching, and deposition, are crucial for developing efficient PICs. Packaging and testing ensure the functional integrity and reliability of PICs.
- Applications of PICs: PICs are transforming telecommunications and data centers by enabling high-speed data transmission and efficient optical interconnects. They are revolutionizing medical and environmental sensing and imaging technologies and hold great potential in emerging fields like photonic computing and quantum technologies.
-
Market Trends and Future Prospects: The current market scenario shows significant growth in PICs, with major players driving innovation. Future trends indicate higher integration levels, advancements in silicon photonics, quantum photonics, and 3D integration. Despite technical and market challenges, opportunities for growth are abundant, driven by expanding applications and collaborative research efforts.
Forward-Looking Perspective
Evolution and Future Potential of PICs
The future of photonic integrated circuits is bright, with numerous advancements and breakthroughs on the horizon:
- Higher Integration and Miniaturization: As fabrication technologies continue to improve, we can expect even higher levels of integration, packing more photonic components onto smaller chips. This will lead to more compact, efficient, and cost-effective solutions, further driving the adoption of PICs across various industries.
- Advanced Material Platforms: Research into new materials and hybrid integration strategies will enhance the performance and functionality of PICs. Materials like indium phosphide (InP), silicon nitride, and emerging 2D materials will play crucial roles in overcoming current limitations and expanding the capabilities of PICs.
- Quantum Photonics: The integration of quantum technologies with PICs promises to revolutionize computing, communication, and sensing. Photonic quantum circuits could lead to practical quantum computers and ultra-secure quantum communication networks, pushing the boundaries of what is technologically possible.
- Photonic Computing: As electronic components approach their physical limits, photonic computing offers a viable path forward. PICs will be central to developing optical processors and neuromorphic computing systems that mimic the human brain, offering unprecedented processing speeds and energy efficiency.
- Broader Applications: The versatility of PICs will lead to their adoption in a wider range of applications. In addition to telecommunications, data centers, medical, and environmental technologies, PICs will find use in autonomous vehicles, smart cities, augmented reality, and more.
-
Standardization and Collaboration: The development of industry standards and increased collaboration between academia, industry, and government will facilitate the widespread adoption of PICs. Standardization will ensure interoperability and compatibility, while collaborative efforts will drive innovation and reduce development costs.
Impact on Society and Industry
The impact of PICs on society and industry will be profound. They will enable faster, more efficient communication networks, enhance medical diagnostics and treatment, improve environmental monitoring, and pave the way for next-generation computing and quantum technologies. As PIC technology continues to evolve, it will play a pivotal role in addressing global challenges and driving economic growth.