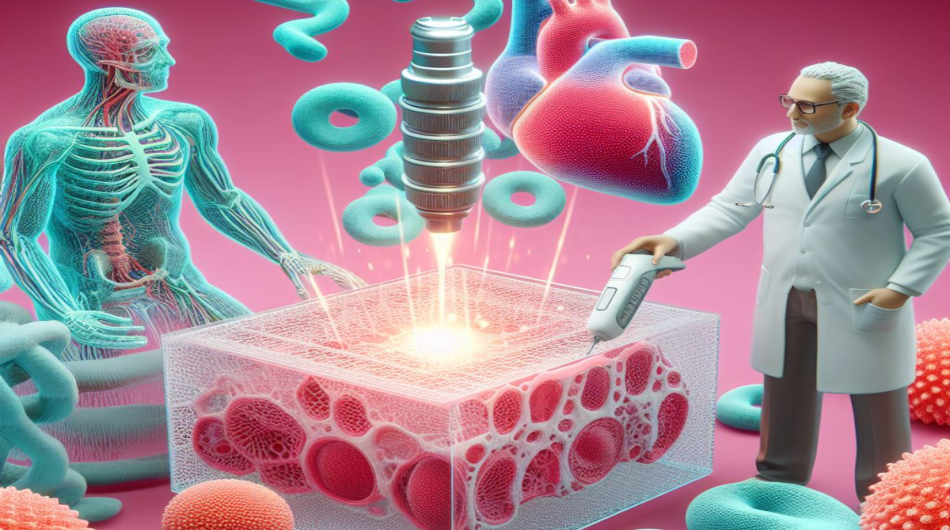
Definition of 3D Printed Tissues
3D printed tissues refer to the fabrication of biological structures using additive manufacturing techniques, where living cells are deposited layer by layer to create complex tissue architectures. This innovative approach enables the precise positioning of cells and biomaterials to replicate the microstructure and functionality of native tissues and organs. 3D printed tissues hold immense potential for applications in regenerative medicine, tissue engineering, and drug discovery, offering new avenues for personalized healthcare and disease treatment.
Overview of 3D Bioprinting Technology
3D bioprinting technology combines principles of engineering, biology, and materials science to create living tissues and organs in a controlled laboratory environment. The process involves three main components: bioinks, bioprinters, and supportive scaffolds. Bioinks serve as the building blocks of tissue, consisting of living cells suspended in a biomaterial matrix. Bioprinters are specialized printers equipped with multiple print heads that deposit bioinks layer by layer to create three-dimensional tissue constructs. Supportive scaffolds provide structural support and guidance for cell growth and tissue development. By precisely controlling the spatial arrangement of cells and biomaterials, 3D bioprinting technology enables the creation of functional tissues and organs with customized properties and functionalities.
Importance and Relevance of 3D Printed Tissues
The development of 3D printed tissues has significant implications for various fields, including regenerative medicine, drug discovery, and personalized healthcare. By providing a platform for fabricating living tissues and organs, 3D bioprinting technology offers new solutions for addressing critical healthcare challenges, such as organ shortage, tissue damage, and drug testing. 3D printed tissues can be tailored to match the specific anatomical and physiological characteristics of individual patients, enabling personalized treatment strategies and reducing the risk of immune rejection. Moreover, 3D bioprinting technology has the potential to revolutionize the field of drug discovery by providing more accurate and physiologically relevant models for testing new drugs and therapies. As such, 3D printed tissues represent a cutting-edge approach to tissue engineering and regenerative medicine, with far-reaching implications for improving human health and advancing medical science.
Historical Background
Early Development of 3D Bioprinting
The roots of 3D bioprinting can be traced back to the early 2000s when researchers began exploring the possibility of using additive manufacturing techniques to create biological structures. One of the earliest demonstrations of 3D bioprinting occurred in 2003 when scientists successfully printed a three-dimensional tube-like structure composed of living cells. This pioneering work laid the foundation for subsequent advancements in the field of tissue engineering and regenerative medicine.
Milestones in 3D Printed Tissues Development
Several key milestones have marked the evolution of 3D printed tissues development over the past two decades. In 2007, researchers at Wake Forest Institute for Regenerative Medicine (WFIRM) made headlines by successfully printing the first functional human organ, a miniature kidney, using a combination of cells and biomaterials. This groundbreaking achievement demonstrated the potential of 3D bioprinting technology to fabricate complex, multicellular tissues with precise architectural control.
Since then, the field of 3D printed tissues has witnessed rapid progress, with researchers achieving significant milestones such as the printing of vascularized tissues, including blood vessels and capillaries, and the creation of functional tissue models for drug screening and disease modeling. These advancements have propelled the field forward and paved the way for the development of more sophisticated bioprinting techniques and applications.
Evolution of Bioprinting Techniques
The evolution of bioprinting techniques has been characterized by the refinement of printing methods, bioink formulations, and scaffold designs to improve the fidelity, viability, and functionality of printed tissues. Early bioprinting approaches relied on extrusion-based and inkjet-based printing methods, which deposited cell-laden bioinks onto a substrate to create three-dimensional structures. While these techniques offered a proof-of-concept for 3D bioprinting, they were limited in their ability to create complex tissue architectures and maintain cell viability over time.
In recent years, advances in bioprinting technology have led to the development of more sophisticated printing platforms, such as laser-based and stereolithography-based bioprinters, which offer higher resolution and precision in printing cellular structures. Additionally, the emergence of novel biomaterials, such as hydrogels and decellularized extracellular matrices, has provided new options for creating bioinks with tailored mechanical and biochemical properties. These advancements have expanded the capabilities of 3D bioprinting technology and unlocked new possibilities for fabricating functional tissues and organs for medical applications.
Science Behind 3D Bioprinting
Cellular Materials Used in Bioprinting
The success of 3D bioprinting relies on the careful selection and manipulation of cellular materials used in the printing process. Various types of cells, including stem cells, primary cells, and cell lines, can be incorporated into bioinks to create tissue constructs with specific functionalities. These cells are often derived from the patient's own tissues or from compatible donor sources to minimize the risk of immune rejection. Depending on the desired tissue type, different cell types are selected and cultured in vitro to achieve the desired phenotype and behavior before being incorporated into bioinks for printing.
Printing Techniques and Methods
3D bioprinting encompasses a diverse range of printing techniques and methods, each with its own advantages and limitations. Some of the most commonly used bioprinting techniques include:
Extrusion-based Bioprinting: This method involves extruding cell-laden bioinks through a nozzle to deposit layers of cells onto a substrate. Extrusion-based bioprinting offers high throughput and versatility, making it suitable for printing large-scale tissue constructs.
Inkjet-based Bioprinting: Inkjet-based bioprinting utilizes piezoelectric or thermal printheads to dispense droplets of bioink onto a substrate. This approach allows for precise control over droplet size and placement, enabling the creation of complex tissue architectures with high resolution.
Laser-based Bioprinting: Laser-based bioprinting utilizes lasers to precisely pattern and deposit cells onto a substrate. This technique offers high spatial resolution and the ability to print cells with subcellular precision, making it ideal for creating intricate tissue structures.
Stereolithography-based Bioprinting: Stereolithography-based bioprinting uses photopolymerization to solidify layers of bioinks in a precise pattern. This method enables the fabrication of three-dimensional tissue constructs with high resolution and fidelity.
Bioinks and Scaffold Materials
Bioinks play a critical role in 3D bioprinting by providing the structural support and biochemical cues necessary for cell growth and tissue development. Bioinks are typically composed of biocompatible polymers, such as hydrogels, alginate, collagen, or gelatin, which mimic the extracellular matrix of native tissues. These biomaterials provide a scaffold for cells to adhere to and organize into functional tissue structures. Additionally, bioinks may contain growth factors, signaling molecules, and other bioactive components to promote cell proliferation, differentiation, and tissue maturation. Scaffold materials are carefully selected based on their mechanical properties, degradation kinetics, and biocompatibility to ensure optimal performance in bioprinting applications.
Applications of 3D Printed Tissues
Regenerative Medicine and Tissue Engineering
One of the most promising applications of 3D printed tissues is in regenerative medicine and tissue engineering. By harnessing the power of bioprinting technology, researchers can fabricate living tissue constructs that closely mimic the structure and function of native tissues and organs. These bioengineered tissues can be used to repair or replace damaged tissues in patients suffering from injuries, diseases, or congenital defects. Examples of tissue-engineered constructs include skin grafts for wound healing, cartilage implants for joint repair, and blood vessel scaffolds for vascular regeneration. 3D printed tissues offer a personalized approach to regenerative medicine, allowing for the creation of patient-specific tissue constructs tailored to individual anatomical and physiological requirements.
Drug Testing and Disease Modeling
Another important application of 3D printed tissues is in drug testing and disease modeling. Traditional two-dimensional cell culture models have limitations in accurately recapitulating the complex microenvironment and physiological responses of native tissues. 3D bioprinting technology enables the fabrication of three-dimensional tissue constructs that more closely resemble the in vivo architecture and function of human tissues. These tissue models can be used to screen drug candidates for efficacy and toxicity, predict patient-specific responses to therapeutics, and study the underlying mechanisms of disease progression. By providing more physiologically relevant models for drug testing and disease modeling, 3D printed tissues have the potential to accelerate the drug discovery process, reduce the reliance on animal testing, and improve patient outcomes.
Personalized Medicine and Organ Transplantation
3D printed tissues hold promise for advancing personalized medicine and organ transplantation by providing customized solutions for patients in need of organ replacement or repair. With advancements in bioprinting technology, researchers can fabricate complex organ structures, such as kidneys, livers, and hearts, using patient-specific cells and biomaterials. These bioengineered organs can be used for transplantation or as in vitro models for drug testing and disease research. Additionally, 3D printed tissues offer the potential to overcome the limitations of organ transplantation, such as donor scarcity, organ rejection, and long waiting times. By enabling the fabrication of patient-specific tissues and organs, 3D bioprinting technology has the potential to revolutionize the field of organ transplantation and usher in a new era of personalized medicine.
Advantages and Challenges
Advantages of 3D Bioprinting Technology
3D bioprinting technology offers several advantages over traditional tissue engineering and regenerative medicine approaches:
Precision and Control: Bioprinting allows for precise control over the spatial arrangement of cells and biomaterials, enabling the creation of complex tissue architectures with high fidelity.
Customization and Personalization: Bioprinting enables the fabrication of patient-specific tissue constructs tailored to individual anatomical and physiological requirements, facilitating personalized treatment strategies.Scalability and Reproducibility: Bioprinting technology can be scaled up for mass production of tissue constructs, offering a cost-effective and reproducible solution for tissue engineering applications.
Integration of Multiple Cell Types: Bioprinting enables the incorporation of multiple cell types within tissue constructs, mimicking the heterogeneity and complexity of native tissues and organs.
Reduction of Animal Testing: Bioprinted tissue models provide more physiologically relevant alternatives to animal testing for drug screening and disease modeling, reducing the reliance on animal models in biomedical research.
Challenges and Limitations
Despite its potential, 3D bioprinting technology faces several challenges and limitations:
Biocompatibility and Cell Viability: Ensuring the biocompatibility of biomaterials and the viability of printed cells remains a significant challenge in bioprinting, as inadequate cell survival and functionality can compromise the performance of printed tissues.
Vascularization and Tissue Integration: Achieving adequate vascularization and tissue integration within printed constructs is essential for supporting cell viability, nutrient transport, and tissue maturation. Current bioprinting techniques struggle to replicate the complex vasculature of native tissues, limiting the size and complexity of printed constructs.
Regulatory Hurdles: Bioprinted tissues face regulatory hurdles related to safety, efficacy, and quality control, which must be addressed to ensure their clinical translation and commercialization.
Scalability and Cost: Scaling up bioprinting processes for large-scale tissue production remains a challenge due to the complexity and cost of bioprinting technology, as well as the limited availability of suitable biomaterials and cell sources.
Current Research and Future Directions
Ongoing research in 3D bioprinting is focused on addressing these challenges and advancing the capabilities of bioprinting technology. Some areas of current research and future directions include:
Vascularization Strategies: Developing innovative approaches for vascularization, such as biofabrication of prevascularized tissue constructs and integration of microfluidic channels within bioprinted tissues.
Biomaterial Development: Designing novel biomaterials with tunable mechanical, biochemical, and degradation properties to better mimic the extracellular matrix of native tissues and enhance tissue integration.
Multi-material Bioprinting: Advancing multi-material bioprinting techniques to enable the incorporation of multiple cell types, growth factors, and signaling molecules within tissue constructs for enhanced functionality and complexity.
Clinical Translation: Conducting preclinical studies and clinical trials to evaluate the safety and efficacy of bioprinted tissues for various applications, such as tissue repair, organ transplantation, and drug testing.
Automation and Scalability: Implementing automated bioprinting platforms and high-throughput screening techniques to increase the efficiency, scalability, and reproducibility of bioprinting processes.
By addressing these challenges and advancing the capabilities of bioprinting technology, researchers aim to realize the full potential of 3D bioprinted tissues for regenerative medicine, drug discovery, and personalized healthcare.
Regulatory Considerations
FDA and Regulatory Oversight
The Food and Drug Administration (FDA) plays a critical role in regulating 3D bioprinted tissues and associated products in the United States. Bioprinted tissues intended for clinical use are subject to FDA oversight and must undergo rigorous preclinical testing and regulatory review to ensure safety, efficacy, and quality. The FDA evaluates bioprinted tissues as medical devices or biologics, depending on their intended use and characteristics. Manufacturers are required to demonstrate compliance with FDA regulations, including good manufacturing practices (GMP), quality control standards, and labeling requirements. FDA approval or clearance is necessary before bioprinted tissues can be marketed and distributed for clinical use.
Ethical Implications and Guidelines
The development and application of 3D bioprinting technology raise important ethical considerations related to patient consent, privacy, equity, and social responsibility. Ethical guidelines and principles play a crucial role in guiding the responsible conduct of research and ensuring the ethical use of bioprinted tissues. Researchers and practitioners are encouraged to adhere to ethical standards, such as respect for autonomy, beneficence, nonmaleficence, and justice, in all aspects of bioprinting research and practice. Ethical review boards and institutional review committees oversee research involving human subjects and ensure compliance with ethical guidelines and regulations. Additionally, stakeholders are encouraged to engage in dialogue and consultation with diverse perspectives to address ethical challenges and promote ethical decision-making in bioprinting research and applications.
International Regulations and Harmonization Efforts
In addition to domestic regulations, 3D bioprinted tissues are subject to international regulations and harmonization efforts aimed at ensuring consistency and coherence in regulatory standards across countries and regions. International organizations, such as the World Health Organization (WHO) and the International Organization for Standardization (ISO), play a role in developing harmonized guidelines and standards for the evaluation and regulation of bioprinted tissues. Efforts to harmonize regulations aim to streamline regulatory processes, facilitate international collaboration and trade, and promote global access to safe and effective bioprinted tissues. However, challenges remain in achieving harmonization due to differences in regulatory frameworks, cultural norms, and healthcare systems among countries. Continued collaboration and coordination among regulatory agencies, industry stakeholders, and international organizations are essential for advancing regulatory harmonization efforts and ensuring the responsible development and use of bioprinted tissues on a global scale.
Commercialization and Industry Landscape
Leading Companies and Research Institutions
The field of 3D bioprinting is characterized by a diverse landscape of companies and research institutions at the forefront of innovation and commercialization. Leading companies and research institutions in the 3D bioprinting space include:
Organovo: Organovo is a biotechnology company that specializes in 3D bioprinting of human tissues for drug discovery and preclinical testing applications.
CELLINK: CELLINK is a bioprinting company that offers a range of bioprinters, bioinks, and software solutions for tissue engineering and regenerative medicine applications.
RegenHU: RegenHU is a Swiss-based company that develops bioprinting platforms and biofabrication technologies for research and clinical applications
Wake Forest Institute for Regenerative Medicine (WFIRM): WFIRM is a leading research institution in the field of regenerative medicine and tissue engineering, with expertise in 3D bioprinting of tissues and organs.
Harvard University Wyss Institute: The Wyss Institute at Harvard University is a multidisciplinary research institute that pioneers innovative bioprinting technologies and approaches for biomedical applications.
Market Trends and Opportunities
The market for 3D bioprinted tissues is experiencing rapid growth and expansion, driven by increasing demand for personalized medicine, regenerative therapies, and drug discovery solutions. Key market trends and opportunities in the 3D bioprinting industry include:
Growing Investment and Funding: The 3D bioprinting industry has attracted significant investment and funding from venture capitalists, government agencies, and private investors, fueling research and development efforts and driving market growth.
Expansion of Applications: The applications of 3D bioprinted tissues are expanding beyond regenerative medicine to include drug testing, disease modeling, personalized medicine, and organ transplantation, creating new opportunities for market growth and innovation.
Technological Advancements: Ongoing advancements in bioprinting technology, materials science, and tissue engineering are driving innovation and improving the performance, scalability, and reproducibility of bioprinted tissues, opening up new possibilities for commercialization and market adoption.
Emerging Markets: Emerging markets, particularly in Asia-Pacific and Latin America, are experiencing rapid growth in the adoption of 3D bioprinting technologies, driven by increasing healthcare spending, rising prevalence of chronic diseases, and growing demand for regenerative therapies.
Challenges in Scaling Production and Market Adoption
Despite the significant opportunities in the 3D bioprinting market, several challenges remain in scaling production and achieving widespread market adoption:
Cost and Affordability: The high cost of bioprinting equipment, materials, and technologies poses a barrier to adoption, particularly for small and medium-sized enterprises (SMEs) and healthcare providers with limited financial resources.
Regulatory Hurdles: Regulatory hurdles and compliance requirements, such as FDA approval, CE marking, and ISO certification, can be time-consuming and costly, delaying market entry and commercialization efforts.
Technical Complexity: Bioprinting technologies are complex and require specialized expertise in materials science, tissue engineering, and biomanufacturing, limiting the accessibility and adoption of bioprinting solutions by non-experts.
Standardization and Quality Control: Standardization of bioprinting processes, materials, and quality control measures is essential for ensuring reproducibility, reliability, and safety of bioprinted tissues, but achieving consensus on standards and protocols remains a challenge.
Addressing these challenges will require collaboration and coordination among industry stakeholders, regulatory agencies, academic institutions, and healthcare providers to drive innovation, reduce costs, and accelerate the adoption of 3D bioprinting technologies in clinical practice and commercial markets.
Collaborations and Partnerships
Academia-Industry Collaborations
Academia-industry collaborations play a crucial role in advancing 3D bioprinting technology, facilitating knowledge transfer, and accelerating commercialization efforts. These collaborations bring together academic researchers, who possess expertise in fundamental science and technology development, with industry partners, who contribute resources, infrastructure, and market access. Academia-industry collaborations in the field of 3D bioprinting often involve joint research projects, technology licensing agreements, and collaborative development of products and applications. By leveraging complementary strengths and capabilities, academia-industry collaborations drive innovation, address technical challenges, and translate research discoveries into commercial products and solutions.
Public-Private Partnerships
Public-private partnerships (PPPs) bring together government agencies, research institutions, and industry stakeholders to address common challenges, promote innovation, and stimulate economic growth. In the context of 3D bioprinting, PPPs support collaborative research and development initiatives, provide funding and resources for technology development, and facilitate technology transfer and commercialization efforts. PPPs may involve government funding agencies, such as the National Institutes of Health (NIH) or the National Science Foundation (NSF), collaborating with industry partners to support research projects, infrastructure development, and workforce training programs. By fostering collaboration and coordination among diverse stakeholders, PPPs accelerate the translation of 3D bioprinting technologies from the laboratory to the marketplace, driving innovation and societal impact.
Collaborative Research Initiatives
Collaborative research initiatives bring together multidisciplinary teams of scientists, engineers, clinicians, and industry experts to tackle complex challenges and advance the state-of-the-art in 3D bioprinting. These initiatives may be initiated by government agencies, research institutions, or industry consortia and often focus on specific areas of research, such as tissue engineering, regenerative medicine, or drug discovery. Collaborative research initiatives may involve joint research projects, shared infrastructure and resources, and collaborative publication and dissemination of research findings. By fostering collaboration and knowledge exchange among researchers and practitioners, collaborative research initiatives drive innovation, accelerate technology development, and enhance the competitiveness of the 3D bioprinting field.
Overall, collaborations and partnerships are essential for advancing 3D bioprinting technology, promoting innovation, and driving commercialization efforts. By bringing together diverse expertise, resources, and perspectives, collaborations and partnerships facilitate the translation of research discoveries into real-world applications, ultimately benefiting patients, healthcare providers, and society as a whole. Continued investment in collaborative initiatives and partnerships will be essential for realizing the full potential of 3D bioprinting and its impact on healthcare and biomedical research.
Public Perception and Education
Consumer Awareness and Acceptance
Consumer awareness and acceptance of 3D bioprinted tissues play a crucial role in shaping the adoption and uptake of bioprinting technologies in healthcare and biomedical research. Public perception of bioprinting is influenced by factors such as familiarity with the technology, understanding of its potential benefits and risks, and trust in regulatory oversight and safety standards. Efforts to increase consumer awareness and acceptance of 3D bioprinted tissues include:
Educational Campaigns: Public education campaigns aim to raise awareness of 3D bioprinting technology, its applications, and its potential impact on healthcare and society. These campaigns use various channels, such as social media, websites, and educational materials, to disseminate information and engage the public in dialogue about bioprinting.
Media Coverage: Media coverage of bioprinting research and advancements can influence public perception by providing information, generating interest, and shaping narratives about the technology. Positive media coverage highlighting the potential benefits of bioprinted tissues for regenerative medicine, drug discovery, and personalized healthcare can help build public trust and acceptance.
Patient Advocacy Groups: Patient advocacy groups and organizations play a key role in raising awareness of bioprinting technology among patient communities, advocating for research funding, and providing support and resources for individuals affected by conditions that may benefit from bioprinted tissues.
Education and Outreach Programs
Education and outreach programs are essential for building public understanding of 3D bioprinting technology, fostering interest in STEM (science, technology, engineering, and mathematics) fields, and promoting career opportunities in biotechnology and biomedical engineering. These programs target students, educators, and the general public and aim to:
STEM Education: STEM education initiatives introduce students to the principles of bioprinting, tissue engineering, and regenerative medicine through hands-on activities, workshops, and educational resources. These programs inspire interest in STEM fields and prepare the next generation of scientists, engineers, and healthcare professionals to innovate in bioprinting technology.
Public Engagement Events: Public engagement events, such as science fairs, open houses, and community workshops, provide opportunities for researchers, educators, and industry professionals to interact with the public, showcase bioprinting technology, and address questions and concerns. These events foster dialogue, promote understanding, and build trust between stakeholders.
Professional Development: Professional development programs for educators, healthcare professionals, and industry professionals provide training and resources to support the integration of bioprinting technology into curricula, clinical practice, and research initiatives. These programs ensure that stakeholders have the knowledge and skills necessary to effectively utilize bioprinting technology and engage with the public.
Addressing Public Concerns and Misconceptions
Addressing public concerns and misconceptions about 3D bioprinting technology is essential for building trust, ensuring transparency, and fostering informed decision-making. Common concerns and misconceptions include:
Safety and Efficacy: Concerns about the safety and efficacy of bioprinted tissues, including the risk of rejection, contamination, and long-term effects, can influence public perception. Transparent communication about the regulatory oversight, quality control measures, and preclinical testing procedures can help alleviate these concerns.
Ethical Considerations: Ethical concerns about the use of bioprinting technology, such as issues related to consent, equity, and unintended consequences, may arise. Open dialogue and engagement with stakeholders, including ethicists, policymakers, and patient advocates, can help address these concerns and ensure that ethical principles guide the development and use of bioprinted tissues.
Environmental Impact: Questions about the environmental impact of bioprinting technology, such as resource consumption, waste generation, and sustainability, are also important considerations. Efforts to minimize environmental impact through sustainable practices, waste reduction strategies, and life cycle assessments can help address these concerns and promote responsible use of bioprinting technology.
By addressing public concerns and misconceptions and promoting education and awareness of 3D bioprinting technology, stakeholders can foster a positive public perception, build trust, and support the responsible development and use of bioprinted tissues for the benefit of healthcare and society.
Conclusion
Recap of Key Points
In summary, the exploration of 3D printed tissues has illuminated a landscape ripe with potential for revolutionizing various sectors, including healthcare, research, and manufacturing. Throughout this exploration, several key points have emerged:
Technological Advancements: 3D bioprinting technology has undergone significant advancements, enabling the precise fabrication of complex tissue structures with high fidelity and functionality.
Applications: The applications of 3D printed tissues span a wide range, from regenerative medicine and drug testing to personalized medicine and organ transplantation, offering promising solutions to pressing healthcare challenges.
Challenges and Considerations: Despite its promise, 3D bioprinting faces challenges such as regulatory hurdles, technical complexities, and public perceptions that must be addressed to realize its full potential.
Future Outlook for 3D Printed Tissues
Looking ahead, the future outlook for 3D printed tissues is bright and brimming with opportunities. Anticipated developments include:
Technological Advancements: Continued advancements in bioprinting technology, materials science, and tissue engineering are expected to enhance the performance, scalability, and reproducibility of 3D printed tissues, unlocking new possibilities for clinical translation and commercialization.
Expanded Applications: The expansion of applications for 3D printed tissues beyond regenerative medicine to include drug discovery, disease modeling, and personalized healthcare is poised to drive further innovation and market growth in the coming years.
Increased Adoption: With growing investment, collaboration, and public awareness, the adoption of 3D printed tissues is expected to increase, leading to greater accessibility, affordability, and acceptance of bioprinting technology in healthcare and biomedical research.
Final Thoughts on the Impact of Bioprinting Technology
In conclusion, the impact of bioprinting technology on healthcare and society has the potential to be transformative. By harnessing the power of 3D bioprinting, researchers and industry stakeholders can address unmet medical needs, accelerate drug discovery, and advance personalized medicine. However, realizing this potential requires concerted efforts to overcome challenges, foster collaborations, and promote education and awareness. With dedication and innovation, bioprinting technology has the capacity to revolutionize healthcare delivery, improve patient outcomes, and shape the future of medicine for generations to come.