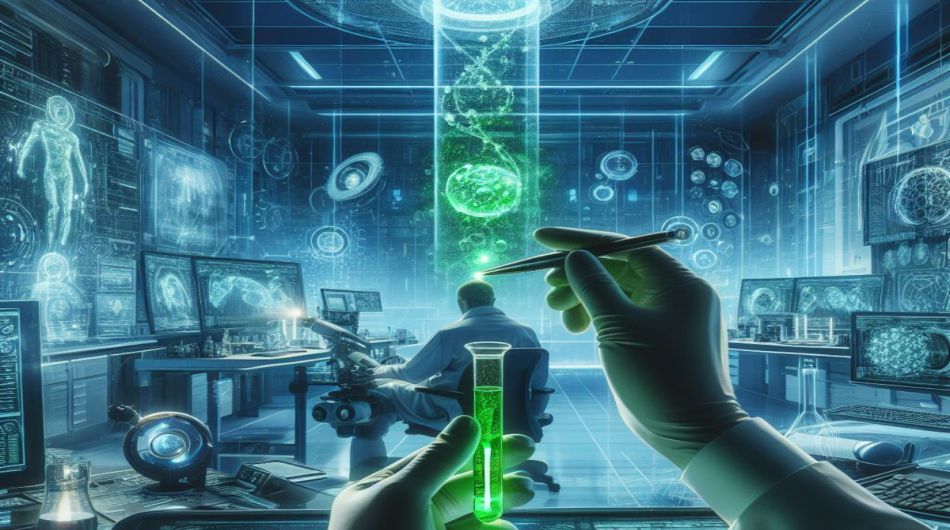
Artificial chlorophyll is a rapidly emerging field that focuses on the development of synthetic analogs of natural chlorophyll, the primary pigment responsible for photosynthesis in plants and other photosynthetic organisms. By mimicking the structure and function of chlorophyll, researchers aim to create artificial systems that can efficiently capture and convert solar energy into chemical energy, with potential applications in renewable energy production, carbon dioxide reduction, and the synthesis of valuable chemicals and fuels. This article provides a comprehensive overview of artificial chlorophyll, including its fundamental principles, key components, synthesis methods, current research progress, challenges, and future prospects.
Fundamental Principles of Artificial Chlorophyll
Artificial chlorophyll draws inspiration from the natural process of photosynthesis, in which chlorophyll plays a central role in harvesting solar energy and converting it into chemical energy. In natural photosynthesis, chlorophyll molecules are organized into light-harvesting complexes and reaction centers, where they absorb photons and transfer the excitation energy to other molecules, ultimately driving the oxidation of water and the reduction of carbon dioxide to produce carbohydrates and oxygen.
The basic principles of artificial chlorophyll involve:
-
Light absorption: Designing synthetic molecules that can efficiently absorb solar energy across a wide range of the visible and near-infrared spectrum.
-
Energy transfer: Facilitating the efficient transfer of excitation energy from the light-absorbing molecules to other components of the artificial photosynthetic system.
-
Charge separation: Promoting the spatial separation of photogenerated electrons and holes to prevent their recombination and enable their use in redox reactions.
-
Catalysis: Coupling the light-absorbing and charge-separating properties of artificial chlorophyll with suitable catalysts to drive the desired chemical reactions, such as water splitting or CO2 reduction.
By optimizing each of these steps and integrating them into a functional system, artificial chlorophyll has the potential to provide a sustainable and scalable solution for harnessing solar energy and producing renewable fuels and chemicals.
Advantages of Artificial Chlorophyll
Artificial chlorophyll offers several key advantages over natural chlorophyll and other artificial photosynthetic systems:
-
Tunability: The structure and properties of artificial chlorophyll molecules can be easily modified through chemical synthesis, allowing for fine-tuning of their light-harvesting, energy transfer, and redox properties.
-
Stability: Artificial chlorophyll molecules can be designed to be more robust and stable than natural chlorophyll, which is prone to degradation under intense light or harsh conditions.
-
Scalability: The synthesis of artificial chlorophyll molecules can be scaled up using established chemical processes, enabling their production in large quantities for practical applications.
-
Versatility: Artificial chlorophyll can be integrated with a wide range of other components, such as catalysts, electrodes, and scaffolds, to create customized artificial photosynthetic systems for specific applications.
Challenges in Artificial Chlorophyll
Despite its potential benefits, the development of efficient and practical artificial chlorophyll systems faces several challenges:
-
Complexity: Natural photosynthesis is a highly complex and regulated process that involves multiple steps and components, making it difficult to replicate artificially.
-
Efficiency: Achieving high quantum yields and energy conversion efficiencies in artificial chlorophyll systems remains a challenge, particularly for the complete process of solar-to-fuel conversion.
-
Stability: Ensuring the long-term stability and durability of artificial chlorophyll systems under operational conditions, such as exposure to light, heat, and moisture, is crucial for their practical application.
-
Cost: Developing cost-effective and scalable methods for the synthesis and assembly of artificial chlorophyll systems is essential for their economic viability and widespread adoption.
Researchers are actively working to address these challenges through a combination of rational design, chemical synthesis, spectroscopic characterization, and device engineering.
Synthesis and Characterization of Artificial Chlorophyll
The synthesis of artificial chlorophyll molecules typically involves the chemical modification of natural chlorophyll or the de novo synthesis of chlorophyll analogs using organic chemistry techniques. The choice of synthesis method depends on the desired structure, properties, and functionality of the artificial chlorophyll molecule.
Modification of Natural Chlorophyll
One approach to creating artificial chlorophyll is to modify the structure of natural chlorophyll molecules through chemical reactions. This can involve the substitution of functional groups, the insertion of metal ions, or the alteration of the porphyrin ring system. Some examples of modified natural chlorophyll include:
-
Chlorin e6: A derivative of chlorophyll a that has been used as a photosensitizer in photodynamic therapy and as a light-harvesting component in artificial photosynthetic systems.
-
Zinc phthalocyanine: A synthetic analog of chlorophyll that contains a central zinc ion instead of magnesium, exhibiting enhanced light absorption and stability.
-
Bacteriochlorophyll derivatives: Modified forms of bacteriochlorophyll, the primary pigment in photosynthetic bacteria, that have been used as light-harvesting and charge-separating components in artificial photosynthetic systems.
De Novo Synthesis of Chlorophyll Analogs
Another approach to creating artificial chlorophyll is to synthesize chlorophyll analogs from scratch using organic chemistry techniques. This allows for greater flexibility in the design and optimization of the molecule's structure and properties. Some examples of de novo synthesized chlorophyll analogs include:
-
Porphyrins: Synthetic porphyrin molecules that mimic the basic structure of chlorophyll but can be functionalized with various substituents to tune their light-harvesting and redox properties.
-
Corroles: Tetrapyrrolic macrocycles that are structurally similar to porphyrins but have a smaller core and exhibit unique photophysical and catalytic properties.
-
BODIPY dyes: Boron-dipyrromethene dyes that are highly fluorescent and have been used as artificial light-harvesting antennas in conjunction with other components, such as porphyrins or fullerenes.
Characterization Techniques
The characterization of artificial chlorophyll molecules involves a range of spectroscopic and analytical techniques to determine their structural, photophysical, and electrochemical properties. Some commonly used characterization techniques include:
-
UV-Vis spectroscopy: Measures the absorption spectrum of the artificial chlorophyll molecule, providing information on its light-harvesting properties and electronic transitions.
-
Fluorescence spectroscopy: Measures the emission spectrum and quantum yield of the artificial chlorophyll molecule, giving insights into its excited-state dynamics and energy transfer processes.
-
Cyclic voltammetry: Determines the redox potentials and electron transfer properties of the artificial chlorophyll molecule, which are crucial for its ability to drive chemical reactions.
-
NMR spectroscopy: Provides detailed information on the molecular structure and conformation of the artificial chlorophyll molecule.
-
Mass spectrometry: Confirms the molecular weight and composition of the artificial chlorophyll molecule.
-
X-ray crystallography: Determines the precise three-dimensional structure of the artificial chlorophyll molecule in the solid state.
These characterization techniques provide valuable information for understanding the structure-property relationships of artificial chlorophyll molecules and guiding their rational design and optimization.
Current Research Progress and Applications
Research on artificial chlorophyll has made significant progress in recent years, with advances in the design, synthesis, and characterization of new molecules and systems. Some notable achievements and applications include:
Light-Harvesting Antennas
Artificial chlorophyll molecules have been used as light-harvesting antennas in artificial photosynthetic systems, mimicking the role of natural chlorophyll in capturing solar energy and transferring it to other components. For example:
-
Porphyrin arrays: Covalently linked arrays of porphyrin molecules have been designed to absorb light over a broad spectral range and funnel the excitation energy to a central reaction center.
-
BODIPY-porphyrin dyads: Conjugates of BODIPY dyes and porphyrins have been synthesized as efficient light-harvesting antennas, exhibiting high absorption coefficients and fast energy transfer rates.
-
Supramolecular assemblies: Non-covalent assemblies of artificial chlorophyll molecules, such as porphyrin-based nanostructures or metal-organic frameworks, have been developed as light-harvesting systems with tunable properties.
Charge Separation and Electron Transfer
Artificial chlorophyll molecules have been combined with electron acceptors and donors to create charge-separating dyads, triads, and more complex systems that mimic the electron transfer processes in natural photosynthesis. For example:
-
Porphyrin-fullerene dyads: Covalently linked porphyrin-fullerene dyads have been synthesized as simple models of photosynthetic reaction centers, exhibiting efficient charge separation and long-lived charge-separated states.
-
Donor-acceptor triads: Artificial chlorophyll molecules have been incorporated into donor-acceptor triads with secondary electron donors and acceptors, achieving multi-step electron transfer cascades and enhanced charge separation lifetimes.
-
Hybrid systems: Artificial chlorophyll molecules have been integrated with inorganic semiconductors, such as TiO2 or carbon nitride, to create hybrid photocatalytic systems for solar fuel production.
Photocatalytic Applications
Artificial chlorophyll molecules have been used as photocatalysts or photosensitizers in various artificial photosynthetic systems for solar fuel production and chemical synthesis. For example:
-
Water splitting: Artificial chlorophyll-based photocatalysts have been developed for the photochemical splitting of water into hydrogen and oxygen, a key step in solar hydrogen production.
-
CO2 reduction: Artificial chlorophyll molecules have been coupled with CO2 reduction catalysts, such as rhenium or cobalt complexes, to photocatalytically convert CO2 into carbon monoxide, formic acid, or other value-added chemicals.
-
Organic synthesis: Artificial chlorophyll photocatalysts have been used in the selective synthesis of organic compounds, such as the oxidation of alcohols or the reduction of alkenes, using solar energy as the driving force.
Biohybrid Systems
Artificial chlorophyll molecules have been integrated with biological components, such as enzymes or whole cells, to create biohybrid systems that combine the advantages of natural and artificial photosynthesis. For example:
-
Enzyme-coupled systems: Artificial chlorophyll photosensitizers have been coupled with hydrogenase or formate dehydrogenase enzymes to achieve light-driven hydrogen evolution or CO2 reduction.
-
Whole-cell systems: Artificial chlorophyll molecules have been introduced into photosynthetic bacteria or algae to enhance their light-harvesting or energy transfer capabilities, improving the efficiency of biological hydrogen or biofuel production.
These examples highlight the diverse applications and potential of artificial chlorophyll in the field of artificial photosynthesis and solar energy conversion. However, there are still significant challenges and opportunities for further development, as discussed in the next section.
Challenges and Future Directions
Despite the significant progress made in artificial chlorophyll research, there are still several challenges and opportunities for future development:
Molecular Design and Optimization
The rational design and optimization of artificial chlorophyll molecules with desired properties, such as broad light absorption, efficient energy transfer, and long-lived charge separation, remain a challenge. Future research directions in this area include:
-
Structure-property relationships: Developing a deeper understanding of the relationship between the molecular structure and photophysical properties of artificial chlorophyll molecules through a combination of experimental and computational studies.
-
Bio-inspired design: Drawing inspiration from the structure and function of natural chlorophyll and other photosynthetic pigments to guide the design of new artificial chlorophyll molecules with improved properties.
-
High-throughput screening: Applying computational and experimental high-throughput screening methods to identify promising artificial chlorophyll candidates from large libraries of molecules.
System Integration and Optimization
Integrating artificial chlorophyll molecules into functional and efficient artificial photosynthetic systems requires careful design and optimization of the various components and interfaces. Future research directions in this area include:
-
Supramolecular assembly: Developing strategies for the controlled self-assembly of artificial chlorophyll molecules into well-defined supramolecular structures with optimized light-harvesting, energy transfer, and charge separation properties.
-
Interfacial engineering: Optimizing the interfaces between artificial chlorophyll molecules and other components, such as catalysts, electrodes, or biological systems, to enhance the efficiency and stability of the overall system.
-
Multiscale modeling: Applying multiscale computational modeling approaches to simulate and optimize the performance of artificial chlorophyll-based systems, from the molecular level to the device level.
Scalability and Sustainability
Demonstrating the scalability and sustainability of artificial chlorophyll-based systems is crucial for their practical implementation and commercialization. Future research directions in this area include:
-
Scalable synthesis: Developing efficient and scalable methods for the synthesis of artificial chlorophyll molecules and their integration into functional materials and devices.
-
Renewable feedstocks: Exploring the use of renewable and abundant feedstocks, such as plant-derived porphyrins or chlorophylls, as starting materials for the synthesis of artificial chlorophyll molecules.
-
Life cycle assessment: Conducting comprehensive life cycle assessments of artificial chlorophyll-based systems to evaluate their environmental impact and sustainability, from raw material extraction to end-of-life disposal.
Innovative Applications
Expanding the application scope of artificial chlorophyll beyond solar energy conversion and exploring new and innovative uses of these molecules is an exciting area for future research. Some potential applications include:
-
Biomedical applications: Investigating the use of artificial chlorophyll molecules as photosensitizers for photodynamic therapy, antimicrobial treatments, or bioimaging.
-
Sensing and detection: Developing artificial chlorophyll-based sensors for the detection of specific molecules, such as pollutants, toxins, or biomarkers, based on their photophysical or photochemical responses.
-
Smart materials: Incorporating artificial chlorophyll molecules into stimuli-responsive or adaptive materials, such as photochromic or photoelectric systems, for applications in energy-efficient buildings, smart windows, or wearable electronics.
By addressing these challenges and opportunities, artificial chlorophyll research has the potential to make significant contributions to the development of sustainable and innovative technologies for energy, environment, and beyond.
Conclusion
Artificial chlorophyll is a fascinating and rapidly growing field that holds great promise for harnessing the power of sunlight to produce renewable fuels, chemicals, and materials. By mimicking and improving upon the natural process of photosynthesis, artificial chlorophyll systems have the potential to provide a sustainable and scalable solution for meeting the world's growing energy demands while reducing our reliance on fossil fuels.
The synthesis and characterization of artificial chlorophyll molecules have made significant progress in recent years, enabling the design of molecules with tunable light-harvesting, energy transfer, and redox properties. These molecules have been integrated into various artificial photosynthetic systems, demonstrating their potential for solar energy conversion, photocatalysis, and biohybrid applications.
However, there are still significant challenges to be addressed in terms of molecular design, system integration, scalability, and sustainability. Ongoing research efforts in these areas, along with the exploration of new and innovative applications, are crucial for realizing the full potential of artificial chlorophyll.
As we strive to transition to a more sustainable and carbon-neutral future, artificial chlorophyll represents a promising avenue for harnessing the abundant and renewable energy of the sun. With continued research and development, artificial chlorophyll-based technologies could play a key role in mitigating climate change, reducing pollution, and creating a more sustainable and equitable world for generations to come.
The field of artificial chlorophyll is still in its early stages, and there is much work to be done to fully understand and optimize these systems. However, the rapid progress made in recent years and the growing interest from the scientific community and industry give cause for optimism. As we continue to unravel the secrets of natural photosynthesis and apply this knowledge to the design of artificial systems, we can look forward to a future in which sunlight becomes our primary source of energy and inspiration.