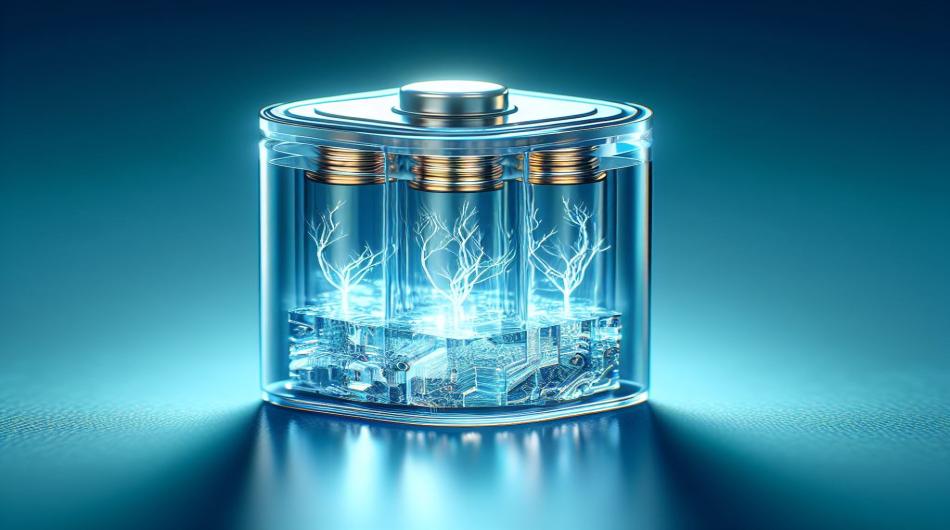
Solid-state batteries are cutting-edge energy storage devices that promise to revolutionize the way we power our world. Unlike traditional lithium-ion batteries, which use liquid electrolytes, solid-state batteries employ solid electrolytes. This fundamental difference opens up a realm of possibilities, from enhanced safety to increased energy density.
In the grand scheme of energy storage solutions, solid-state batteries hold immense importance and relevance. As the demand for cleaner and more sustainable energy sources continues to grow, finding efficient and reliable ways to store this energy becomes paramount. Solid-state batteries offer a promising avenue towards achieving these goals, with their potential to address key challenges faced by current battery technologies.
The history and development of solid-state batteries trace back several decades, with researchers and scientists gradually unravelling their potential. From early experiments with ceramic electrolytes to recent breakthroughs in polymer-based designs, the journey of solid-state batteries is one of continuous innovation and discovery.
Fundamentals of Solid-State Batteries
Basic structure and components
Solid-state batteries comprise three primary components: the electrolyte, cathode, and anode.
-
Electrolyte: The electrolyte in solid-state batteries differs from that in traditional lithium-ion batteries. Instead of a liquid electrolyte, solid-state batteries use a solid electrolyte material, which can be either ceramic or polymer-based. This solid electrolyte serves as the medium for the transport of ions between the cathode and anode.
-
Cathode: The cathode is the positive electrode in a battery, where the reduction reaction occurs during discharge. In solid-state batteries, various materials can be used as cathodes, including oxides, sulfides, and polyanionic compounds. The choice of cathode material influences the battery's performance, energy density, and overall stability.
-
Anode: The anode, on the other hand, is the negative electrode where the oxidation reaction takes place. Similar to the cathode, the anode material plays a crucial role in determining the battery's performance characteristics. Common anode materials include lithium metal, lithium alloys, and carbon-based materials like graphite.
Comparison with traditional lithium-ion batteries
Solid-state batteries offer several advantages over traditional lithium-ion batteries:
-
Safety: Solid electrolytes are non-flammable and less prone to leakage, reducing the risk of thermal runaway and battery fires.
-
Energy density: Solid-state batteries have the potential for higher energy densities compared to conventional lithium-ion batteries, leading to longer driving ranges in electric vehicles and increased runtime in electronic devices.
-
Cycle life: The solid-state electrolyte design can result in longer cycle life and improved durability, reducing the need for frequent battery replacements.
-
Temperature stability: Solid-state batteries exhibit better performance and stability across a wide range of temperatures compared to liquid electrolyte batteries.
Key advantages of solid-state batteries
-
Enhanced safety: Solid-state batteries are inherently safer than traditional lithium-ion batteries due to the absence of flammable liquid electrolytes.
-
Increased energy density: Solid-state batteries have the potential to store more energy in the same volume or weight compared to conventional batteries, enabling longer usage times between charges.
-
Longer cycle life: The use of solid electrolytes can improve the durability and longevity of batteries, leading to fewer replacements and reduced environmental impact.
-
Environmental sustainability: Solid-state batteries can contribute to the transition towards cleaner energy storage solutions, helping to mitigate the environmental impact of fossil fuel consumption.
Fundamental principles of operation
Solid-state batteries operate on the same basic principles as traditional batteries, involving the flow of ions between the cathode and anode during charge and discharge cycles. However, the use of solid electrolytes in place of liquid electrolytes introduces some unique characteristics:
-
Ion transport: Ions move through the solid electrolyte instead of a liquid medium, which can influence the rate of ion transport and overall battery performance.
-
Interface stability: The interface between the solid electrolyte and electrode materials plays a crucial role in determining the battery's efficiency and long-term stability.
-
Electrochemical reactions: The cathode and anode undergo reversible electrochemical reactions during charge and discharge, storing and releasing energy in the form of chemical potential.
Materials and Manufacturing Processes
Electrolyte materials
Solid-state batteries utilize different types of electrolyte materials, each with its own set of properties and advantages.
-
Ceramic: Ceramic electrolytes are commonly used in solid-state batteries due to their high ionic conductivity and chemical stability. Materials such as lithium phosphorus oxynitride (LiPON), lithium lanthanum titanate (LLTO), and lithium garnet (e.g., Li7La3Zr2O12 or LLZO) are examples of ceramic electrolytes. These materials offer excellent mechanical strength and thermal stability, making them suitable for high-performance applications.
-
Polymer: Polymer electrolytes provide flexibility and processability, allowing for easier integration into battery designs. Materials such as polyethene oxide (PEO) and polyvinylidene fluoride (PVDF) are commonly used as polymer electrolytes in solid-state batteries. Polymer electrolytes offer advantages such as improved interface compatibility with electrode materials and enhanced safety due to their non-flammable nature.
Cathode and anode materials
Solid-state batteries employ a variety of cathode and anode materials to enable efficient energy storage and retrieval.
Cathode materials:
-
Transition metal oxides (e.g., lithium cobalt oxide, lithium iron phosphate)
-
Sulfides (e.g., lithium sulfide)
-
Polyanionic compounds (e.g., lithium iron phosphate, lithium manganese phosphate)
Anode materials:
-
Lithium metal
-
Lithium alloys (e.g., lithium silicon, lithium titanium oxide)
-
Carbon-based materials (e.g., graphite, graphene)
The choice of cathode and anode materials depends on factors such as energy density, cycling stability, and cost considerations.
Fabrication methods
Various fabrication methods are employed to manufacture solid-state batteries with precise control over their structure and performance characteristics.
-
Thin-film deposition: Thin-film deposition techniques, such as sputtering and chemical vapour deposition (CVD), are used to deposit thin layers of electrode and electrolyte materials onto substrates. This approach enables the fabrication of uniform and high-quality thin-film solid-state batteries suitable for microelectronics and miniaturized devices.
-
Solid-state electrolyte synthesis: Solid-state electrolytes can be synthesized using techniques such as solid-state sintering, sol-gel processing, and mechanochemical synthesis. These methods allow for the production of dense and homogeneous electrolyte materials with tailored compositions and properties.
-
Electrode fabrication techniques: Electrodes for solid-state batteries are typically fabricated using processes such as tape casting, screen printing, and electrodeposition. These techniques enable the deposition of active materials onto current collectors with precise control over electrode morphology and thickness.
Advancements in Solid-State Battery Technology
Solid-state battery technology has witnessed significant advancements in recent years, driving innovation and pushing the boundaries of energy storage capabilities.
Enhanced energy density
One of the most promising advancements in solid-state battery technology is the achievement of enhanced energy density. By optimizing the design of electrolyte materials and electrode architectures, researchers have been able to increase the amount of energy that can be stored within a given volume or weight of the battery. This improvement translates into longer driving ranges for electric vehicles and extended operating times for portable electronics, addressing one of the key limitations of current battery technologies.
Improved safety features
Safety is a paramount concern in battery technology, especially as the demand for high-energy-density batteries continues to rise. Solid-state batteries offer inherent safety advantages over traditional lithium-ion batteries due to their use of non-flammable solid electrolytes. Recent advancements in solid-state battery technology have further improved safety features by enhancing electrolyte stability, mitigating dendrite formation, and reducing the risk of thermal runaway. These developments not only increase the reliability of solid-state batteries but also contribute to the overall safety of devices and systems powered by these batteries.
Increased cycle life
Cycle life, or the number of charge-discharge cycles a battery can withstand before its capacity significantly degrades, is a critical factor in determining the longevity and economic viability of energy storage systems. Solid-state batteries have demonstrated promising improvements in cycle life compared to conventional lithium-ion batteries. By minimizing electrode degradation, electrolyte decomposition, and other factors that contribute to capacity loss over time, solid-state battery technology has the potential to significantly extend the lifespan of energy storage devices, reducing maintenance costs and environmental impact.
Scalability and commercial viability
Scalability and commercial viability are essential considerations for the widespread adoption of any new technology. Recent advancements in solid-state battery manufacturing processes, such as roll-to-roll production techniques and scalable electrode fabrication methods, have enabled the mass production of solid-state batteries at competitive costs. As manufacturing capabilities continue to improve and economies of scale are realized, solid-state batteries are poised to become increasingly competitive with traditional lithium-ion batteries in terms of cost and performance.
Case studies of recent breakthroughs in solid-state battery research
Several case studies highlight the significant breakthroughs and achievements in solid-state battery research:
-
Development of high-conductivity ceramic electrolytes, such as garnet-based materials, enabling the realization of solid-state batteries with improved performance and safety.
-
Exploration of novel cathode and anode materials, including sulfide-based compounds and lithium metal alloys, to enhance energy density and cycling stability.
-
Integration of advanced manufacturing techniques, such as 3D printing and additive manufacturing, for the rapid prototyping and optimization of solid-state battery designs.
-
Collaboration between academia, industry, and government organizations to accelerate the development and commercialization of solid-state battery technology for various applications, including electric vehicles, grid energy storage, and wearable electronics.
Challenges and Limitations
Despite the promising advancements in solid-state battery technology, several challenges and limitations must be addressed to fully realize their potential.
Cost-effectiveness
One of the primary challenges facing solid-state batteries is their cost-effectiveness. The materials used in solid-state electrolytes, such as high-purity ceramics and specialized polymers, tend to be more expensive than the liquid electrolytes in conventional lithium-ion batteries. Additionally, the manufacturing processes for solid-state batteries are often more complex and costly. To achieve widespread adoption, significant efforts are needed to reduce the production costs through material optimization, scalable manufacturing techniques, and economies of scale.
Scalability issues
Scalability remains a critical hurdle for the commercial viability of solid-state batteries. While laboratory-scale prototypes have demonstrated impressive performance, scaling up these technologies to mass production presents numerous challenges. These include ensuring uniform material properties across larger batches, maintaining high production yields, and integrating solid-state batteries into existing manufacturing infrastructures. Overcoming these scalability issues is essential for transitioning from small-scale production to the large-scale manufacturing required for consumer electronics, electric vehicles, and grid storage.
Interface stability
Interface stability between the solid electrolyte and the electrode materials is a significant concern in solid-state batteries. Poor interface stability can lead to increased resistance, reduced ionic conductivity, and the formation of detrimental interfacial layers, all of which degrade battery performance. Researchers are actively exploring ways to improve interface stability through the development of compatible materials, advanced coating techniques, and innovative engineering solutions. Achieving stable and efficient interfaces is crucial for the long-term reliability and performance of solid-state batteries.
Performance at different temperatures
The performance of solid-state batteries can vary significantly at different temperatures. While solid electrolytes generally exhibit better thermal stability compared to liquid electrolytes, their ionic conductivity can decrease at low temperatures, leading to reduced battery efficiency and power output. Conversely, high temperatures can exacerbate degradation processes and impact the structural integrity of the materials. Developing solid-state batteries that perform reliably across a wide temperature range is essential for applications in diverse environmental conditions, such as automotive and aerospace industries.
Manufacturing complexity
The manufacturing processes for solid-state batteries are inherently more complex than those for traditional lithium-ion batteries. Techniques such as thin-film deposition, solid-state electrolyte synthesis, and precise electrode fabrication require specialized equipment and expertise. This complexity can lead to higher production costs and longer development timelines. Simplifying manufacturing processes, developing standardized procedures, and investing in automation technologies are key strategies to address these challenges and streamline the production of solid-state batteries.
Regulatory hurdles and safety concerns
Regulatory hurdles and safety concerns also pose significant challenges to the widespread adoption of solid-state batteries. Ensuring compliance with stringent safety standards and obtaining regulatory approvals can be time-consuming and costly. While solid-state batteries are inherently safer than their liquid counterparts, rigorous testing and validation are required to address any potential safety issues, such as the mechanical stability of the solid electrolyte and the prevention of dendrite formation. Collaborating with regulatory bodies and establishing comprehensive safety protocols are essential steps to facilitate the market entry of solid-state batteries.
Applications and Future Outlook
Solid-state batteries have the potential to revolutionize various industries by offering superior performance, safety, and longevity. As research and development continue to advance, several key applications and future trends are emerging.
Electric vehicles (EVs)
Electric vehicles (EVs) stand to benefit significantly from solid-state battery technology. The higher energy density of solid-state batteries can translate into longer driving ranges, addressing one of the primary concerns for EV adoption. Additionally, the improved safety features and longer cycle life of solid-state batteries make them highly attractive for automotive applications. Major automakers and battery manufacturers are investing heavily in the development of solid-state batteries, with the goal of deploying them in next-generation EVs. As these advancements materialize, we can expect a substantial increase in EV performance and consumer acceptance.
Consumer electronics
The consumer electronics industry, including smartphones, laptops, and wearable devices, demands batteries that are compact, lightweight, and have high energy densities. Solid-state batteries are well-suited to meet these requirements, providing longer battery life and enhanced safety for portable devices. As solid-state battery technology becomes more commercially viable, we can anticipate its integration into a wide range of consumer electronics, leading to thinner and more powerful gadgets with extended usage times.
Renewable energy storage
Renewable energy storage is another critical area where solid-state batteries can make a significant impact. The intermittent nature of renewable energy sources like solar and wind requires efficient and reliable energy storage solutions. Solid-state batteries offer the potential for higher energy densities and longer cycle lives, making them ideal for grid storage applications. By enhancing the efficiency and stability of energy storage systems, solid-state batteries can facilitate the broader adoption of renewable energy, contributing to a more sustainable and resilient energy infrastructure.
Aerospace and aviation
The aerospace and aviation industries require energy storage solutions that are lightweight, safe, and capable of withstanding extreme conditions. Solid-state batteries meet these criteria, offering superior performance and safety compared to traditional batteries. Potential applications include power systems for electric aircraft, drones, and satellites. The adoption of solid-state batteries in aerospace and aviation could lead to significant advancements in these fields, enabling longer flight times, increased payload capacities, and improved overall efficiency.
Emerging trends and potential disruptive impacts
Several emerging trends and potential disruptive impacts are shaping the future of solid-state battery technology. These include:
-
Integration with advanced materials: The use of novel materials, such as nanostructured electrodes and hybrid electrolytes, can further enhance the performance and stability of solid-state batteries.
-
Fast-charging capabilities: Advances in solid-state battery design may enable ultra-fast charging, significantly reducing the time required to recharge devices and EVs.
-
Flexible and wearable batteries: The development of flexible solid-state batteries opens up new possibilities for wearable electronics and medical devices, providing safe and reliable power sources for a wide range of applications.
-
Sustainability and recycling: As environmental concerns grow, the focus on developing sustainable and recyclable solid-state batteries is increasing, promoting a circular economy in the battery industry.
Predictions for future market penetration and adoption rates
The market penetration and adoption rates of solid-state batteries are expected to grow steadily over the next decade. Initially, high-end and niche applications, such as premium EVs and advanced consumer electronics, will likely lead the adoption. As manufacturing processes become more efficient and cost-effective, solid-state batteries will increasingly replace conventional lithium-ion batteries across various sectors. Analysts predict that by the late 2020s to early 2030s, solid-state batteries could achieve significant market share, driven by their superior performance, safety, and longevity. Collaborative efforts between industry, academia, and government will be crucial in accelerating this transition and overcoming remaining technical and economic challenges.
Conclusion
Summary of key points
Solid-state batteries represent a significant advancement in energy storage technology, offering enhanced energy density, improved safety features, longer cycle life, and greater temperature stability compared to traditional lithium-ion batteries. Key materials such as ceramic and polymer electrolytes, along with innovative fabrication methods, are driving this progress. Despite the many benefits, challenges such as cost-effectiveness, scalability, interface stability, and manufacturing complexity remain.
Reflection on the current state of solid-state battery technology
The current state of solid-state battery technology is marked by rapid advancements and increased interest from various industries. Significant research efforts are being directed towards overcoming existing limitations, with promising breakthroughs in material science and manufacturing processes. While commercial adoption is still in its early stages, the progress made thus far indicates a strong potential for widespread use shortly.
Future prospects and areas for further research
Future prospects for solid-state batteries are promising, with ongoing research focusing on enhancing material properties, improving manufacturing techniques, and ensuring cost-effective scalability. Areas for further research include the development of more stable and conductive solid electrolytes, the optimization of electrode-electrolyte interfaces, and the creation of flexible and high-capacity battery designs. Collaborative efforts between academia, industry, and government will be crucial in accelerating these developments.
Final thoughts on the significance of solid-state batteries in the context of sustainable energy storage solutions
In the context of sustainable energy storage solutions, solid-state batteries hold tremendous significance. They offer the potential to revolutionize various industries, including electric vehicles, consumer electronics, renewable energy storage, and aerospace, by providing safer, more efficient, and longer-lasting power sources. As the world moves towards a more sustainable future, solid-state batteries are poised to play a pivotal role in enabling cleaner energy systems and reducing our reliance on fossil fuels. By continuing to address current challenges and advancing technology, solid-state batteries will be instrumental in achieving a sustainable and energy-efficient future.