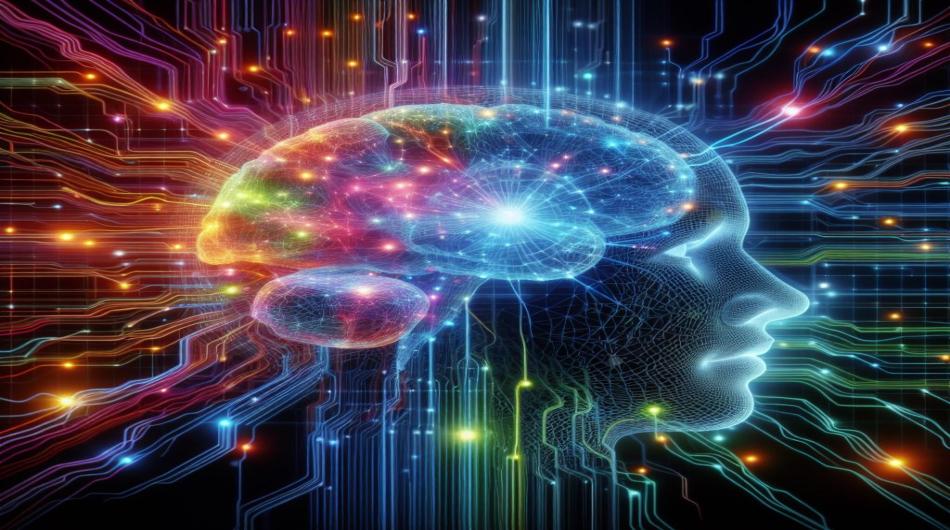
Introduction to Brain-Computer Interfaces (BCIs)
Definition and basic concept
Brain-Computer Interfaces (BCIs) represent an innovative technology that establishes a direct communication pathway between the brain and external devices or computer systems. Essentially, BCIs allow individuals to control and interact with technology using nothing but their thoughts and brain activity.
The basic concept behind BCIs lies in the ability to detect, interpret, and translate neural signals generated by the brain into actionable commands that can be used to control devices or perform tasks. This involves capturing signals such as electrical impulses or changes in blood flow within the brain, processing them using advanced algorithms, and then translating them into meaningful actions or outputs.
Historical overview of BCIs
The roots of BCIs can be traced back to the mid-20th century when researchers began experimenting with techniques to record and analyze brain activity. One of the earliest breakthroughs came in the 1970s when scientists successfully demonstrated the ability to control simple devices using brain signals in animal studies.
Throughout the following decades, advancements in neuroscience, computing, and signal processing led to the development of increasingly sophisticated BCIs. In the 1990s, the first human trials of BCIs were conducted, paving the way for applications in assistive technology, neuroprosthetics, and rehabilitation.
Since then, BCIs have continued to evolve rapidly, with improvements in signal acquisition technology, miniaturization of hardware, and the integration of machine learning algorithms. Today, BCIs are poised to revolutionize various fields, including healthcare, gaming, education, and beyond.
Significance and potential impact of BCIs
The significance of BCIs lies in their potential to transform the way we interact with technology and the world around us. By bypassing traditional input devices such as keyboards or joysticks and tapping directly into the brain's commands, BCIs offer new opportunities for communication, control, and enhancement.
BCIs hold immense promise for individuals with disabilities, offering them greater independence and quality of life. For example, individuals with paralysis can use BCIs to control robotic arms or communicate with others using their thoughts alone. Additionally, BCIs have the potential to revolutionize fields such as neurorehabilitation, virtual reality, and cognitive enhancement, opening up new frontiers for exploration and discovery.
Neurophysiology and Brain Signals
Overview of brain structure and function
The human brain is a remarkably complex organ composed of billions of neurons interconnected through networks of synapses. It is divided into various regions, each responsible for different functions, including perception, cognition, movement, and emotion.
Key structures within the brain include the cerebral cortex, which plays a crucial role in higher cognitive functions such as reasoning and decision-making, and the brainstem, which regulates vital functions such as heartbeat and breathing. Additionally, the brain is divided into lobes, including the frontal, parietal, temporal, and occipital lobes, each associated with specific functions and abilities.
Understanding the structure and function of the brain is essential for interpreting neural signals and developing effective BCIs that can accurately decode and translate brain activity into actionable commands.
Types of brain signals relevant to BCIs
Several types of brain signals are relevant to BCIs, each providing unique insights into brain activity:
-
Electroencephalography (EEG): EEG measures electrical activity in the brain using electrodes placed on the scalp. It is non-invasive and offers high temporal resolution, making it suitable for real-time applications such as controlling assistive devices or monitoring brain states during tasks.
-
Functional Magnetic Resonance Imaging (fMRI): fMRI measures changes in blood flow and oxygenation levels in the brain, providing information about brain activity with high spatial resolution. While fMRI offers detailed anatomical localization of brain activity, it is less suitable for real-time applications due to its slow temporal resolution.
-
Electrocorticography (ECoG): ECoG involves placing electrodes directly on the surface of the brain (the cortex). It provides higher spatial resolution than EEG and can capture more detailed neural activity. ECoG is often used in research settings and clinical applications for studying brain function and controlling neuroprosthetic devices.
Each type of brain signal has its strengths and limitations, and the choice of signal depends on the specific requirements and objectives of the BCI application.
Neural encoding and decoding principles
Neural encoding refers to the process by which information is represented and encoded in the activity of neurons. Different types of neural signals, such as action potentials or local field potentials, encode various aspects of sensory, motor, and cognitive information.
Decoding neural signals involves analyzing and interpreting these signals to extract meaningful information about the user's intentions or mental states. This typically involves the use of signal processing algorithms, machine learning techniques, and pattern recognition algorithms to identify patterns or features in the neural data that correspond to specific commands or actions.
Neural decoding principles vary depending on the type of brain signal being used and the intended application of the BCI. Researchers continue to explore and develop new decoding methods to improve the accuracy, reliability, and speed of BCIs, with the ultimate goal of enabling seamless and intuitive interaction between the brain and external devices or systems.
Types of Brain-Computer Interfaces
Non-invasive BCIs
Non-invasive BCIs utilize external sensors to detect and measure brain activity without the need for surgical implantation. These BCIs are typically more accessible and less invasive than their invasive counterparts, making them suitable for a wide range of applications.
Electroencephalography (EEG):
-
EEG measures electrical activity in the brain using electrodes placed on the scalp.
-
It offers real-time monitoring of brain activity with high temporal resolution, making it suitable for applications such as neurofeedback training, brain-computer interfacing, and monitoring cognitive states.
-
EEG-based BCIs have been used in various fields, including assistive technology, gaming, and neurofeedback therapy.
Functional Near-Infrared Spectroscopy (fNIRS):
-
fNIRS measures changes in blood oxygenation levels in the brain using near-infrared light.
-
It provides a non-invasive method for monitoring brain activity with good spatial resolution, allowing researchers to localize brain activation patterns during cognitive tasks.
-
fNIRS-based BCIs have been explored for applications such as mental workload assessment, brain-computer interfacing, and neurorehabilitation.
Magnetoencephalography (MEG):
-
MEG measures magnetic fields generated by neural activity in the brain.
-
It offers high spatial and temporal resolution, allowing researchers to map brain activity with precision.
-
MEG-based BCIs are primarily used in research settings for studying brain function, cognitive processes, and neurological disorders.
Invasive BCIs
Invasive BCIs involve the surgical implantation of electrodes directly into the brain tissue or onto the surface of the cortex. While more invasive than non-invasive BCIs, invasive BCIs offer higher spatial resolution and signal quality, making them suitable for precise control and communication applications.
Intracortical Electrodes:
-
Intracortical electrodes are implanted into the brain tissue, allowing for direct recording of individual neurons' electrical activity.
-
They offer high spatial and temporal resolution, enabling precise control of prosthetic limbs, communication devices, and other assistive technologies.
-
Intracortical BCIs have shown promising results in restoring motor function and communication abilities in individuals with paralysis or severe disabilities.
Electrocorticography (ECoG):
-
ECoG involves placing electrodes directly on the surface of the brain (the cortex).
-
It provides high-quality signals with excellent spatial resolution, making it suitable for decoding complex neural patterns.
-
ECoG-based BCIs are used primarily in research and clinical settings for studying brain function, epilepsy monitoring, and controlling neuroprosthetic devices.
Hybrid BCIs
Hybrid BCIs combine multiple modalities or techniques to improve performance and overcome limitations associated with individual approaches. By leveraging the strengths of different BCI technologies, hybrid BCIs offer enhanced functionality and versatility for a wide range of applications.
Combining Multiple Modalities:
-
Hybrid BCIs may combine non-invasive and invasive modalities, such as EEG and intracortical electrodes, to capture complementary aspects of brain activity.
-
By integrating multiple signals, hybrid BCIs can achieve higher accuracy, robustness, and reliability compared to single-modality approaches.
-
Hybrid BCIs have been explored for applications such as neuroprosthetics, brain-computer interfacing, and cognitive augmentation, offering new opportunities for enhancing human-machine interaction and communication.
Applications of Brain-Computer Interfaces
Assistive Technology
Communication aids for individuals with disabilities:
-
BCIs enable individuals with severe motor impairments, such as paralysis or locked-in syndrome, to communicate with others using their thoughts alone. By translating neural signals into text or speech output, BCIs provide a lifeline for individuals who are unable to speak or move voluntarily.
Control of prosthetic limbs and exoskeletons:
-
BCIs offer individuals with limb loss or limb paralysis the ability to control prosthetic limbs or exoskeletons using neural signals. By decoding intentions for movement from the brain, BCIs allow users to perform complex tasks such as grasping objects, walking, or manipulating tools with precision and naturalness.
Environmental control systems:
-
BCIs enable individuals with severe physical disabilities to interact with their environment and perform everyday tasks independently. By controlling household appliances, electronic devices, or smart home systems using brain signals, BCIs empower users to maintain autonomy and improve their quality of life.
Neurorehabilitation
Stroke rehabilitation:
-
BCIs play a vital role in stroke rehabilitation by providing real-time feedback and assistance during motor rehabilitation exercises. By monitoring brain activity associated with movement, BCIs can detect and reinforce neural signals related to motor function, facilitating recovery and relearning of motor skills.
Spinal cord injury rehabilitation:
-
BCIs offer hope for individuals with spinal cord injuries by restoring mobility and independence through the control of assistive devices. By bypassing damaged spinal cord pathways and directly activating muscles or prosthetic limbs, BCIs enable individuals to regain movement and functionality lost due to injury.
Cognitive rehabilitation for traumatic brain injury:
-
BCIs are being explored as tools for cognitive rehabilitation in individuals with traumatic brain injury or cognitive impairments. By providing neurofeedback and cognitive training exercises, BCIs can improve attention, memory, and executive function, enhancing cognitive abilities and facilitating recovery.
Augmented and Virtual Reality
Immersive gaming experiences:
-
BCIs enhance gaming experiences by allowing users to control gameplay elements using their thoughts or mental states. By detecting neural signals associated with attention, emotion, or intention, BCIs can adapt game dynamics in real-time, creating immersive and personalized gaming experiences.
Training simulations for medical and military applications:
-
BCIs are used in training simulations for medical professionals and military personnel to improve decision-making, situational awareness, and performance under pressure. By monitoring brain activity, BCIs can assess cognitive load, stress levels, and skill proficiency, providing valuable feedback for training and performance optimization.
Virtual navigation and manipulation of objects:
-
BCIs enable users to interact with virtual environments and manipulate virtual objects using their thoughts. By decoding neural signals related to movement or intention, BCIs allow users to navigate virtual spaces, manipulate virtual objects, and interact with virtual interfaces, enhancing immersion and usability in virtual reality environments.
Cognitive Enhancement
Neurofeedback for cognitive training:
-
BCIs offer neurofeedback training programs that enable individuals to regulate and optimize their cognitive states, such as attention or relaxation. By providing real-time feedback on brain activity, BCIs help individuals develop self-awareness and self-regulation skills, improving cognitive performance and well-being.
Attention and concentration enhancement:
-
BCIs can assist individuals in maintaining focus and attention during tasks by providing feedback on their brain activity patterns. By detecting fluctuations in attention levels, BCIs can prompt users to refocus or adjust their behavior, improving concentration and task performance.
Memory augmentation techniques:
-
BCIs hold promise for enhancing memory function through techniques such as memory encoding or retrieval assistance. By stimulating specific brain regions or providing cues based on neural activity patterns, BCIs can boost memory formation, retention, and recall, aiding individuals in learning and information processing tasks.
Challenges and Limitations of BCIs
Technical challenges
-
Signal processing: Processing and interpreting neural signals captured by BCIs pose significant technical challenges. Neural signals are often weak and susceptible to noise, making it challenging to extract meaningful information reliably. Advanced signal processing algorithms are required to enhance signal quality, remove artifacts, and decode neural activity accurately.
-
Noise reduction: Neural signals obtained from BCIs can be contaminated by various sources of noise, including environmental interference, muscle activity, and electrode artifacts. Developing robust noise reduction techniques is essential to improve signal-to-noise ratio and enhance the reliability and accuracy of BCIs.
-
Feature extraction and classification: Identifying relevant features and patterns in neural data and classifying them into meaningful categories (e.g., motor intentions, cognitive states) is a complex and computationally intensive task. Machine learning algorithms are commonly employed to extract features and train classifiers, but challenges remain in designing algorithms that generalize well across users and tasks.
Ethical considerations
-
Privacy: BCIs raise significant privacy concerns related to the collection, storage, and sharing of sensitive brain data. Ensuring the confidentiality and security of neural data is crucial to protect users' privacy rights and prevent unauthorized access or misuse of their personal information.
-
Consent: Obtaining informed consent from BCI users is essential to ensure autonomy and respect for individual rights. Given the invasive nature of some BCI technologies, such as invasive electrodes, obtaining voluntary and informed consent becomes even more critical to safeguard users' autonomy and well-being.
-
Ownership and control: BCIs raise questions about ownership and control of neural data and the potential for external manipulation or coercion. Clear policies and regulations are needed to address issues of data ownership, control, and usage rights, empowering users to make informed decisions about their data and ensuring accountability and transparency in BCI research and development.
Practical limitations
-
Cost: BCIs often involve expensive equipment and specialized hardware, making them inaccessible to many individuals, particularly those from low-income or marginalized communities. Addressing cost barriers and improving affordability is essential to promote equitable access to BCI technology and ensure that its benefits reach a broader population.
-
Accessibility: BCIs may present accessibility challenges for individuals with physical or cognitive disabilities, particularly those with limited dexterity or cognitive impairments. Designing user-friendly interfaces and accommodating diverse user needs are essential to enhance accessibility and usability of BCIs for individuals with disabilities.
-
Integration and compatibility: Integrating BCIs into existing systems and technologies, such as assistive devices or virtual reality platforms, can be challenging due to compatibility issues and interoperability constraints. Standardization efforts and collaboration among stakeholders are needed to streamline integration processes and ensure seamless interaction between BCIs and external devices or systems.
Future Directions and Emerging Trends
Advances in neural decoding algorithms
-
Deep learning approaches: Future advancements in neural decoding algorithms are likely to leverage deep learning techniques to improve the accuracy and robustness of BCIs. Deep neural networks have shown promise in decoding complex patterns of neural activity and learning representations directly from raw brain signals, enhancing BCI performance across various tasks and applications.
-
Adaptive decoding strategies: Researchers are exploring adaptive decoding strategies that dynamically adjust to changes in neural activity patterns or user intentions. By continuously updating decoding models based on real-time feedback, adaptive BCIs can adapt to individual users' evolving needs and preferences, improving performance and usability over time.
Miniaturization and wearability of BCI devices
-
Wearable BCI technology: The miniaturization and integration of BCI components into wearable devices, such as headsets, smart glasses, or wearable sensors, will enhance portability and accessibility. Wearable BCIs offer users greater freedom of movement and flexibility in interacting with technology, enabling seamless integration into daily life activities and environments.
-
Implantable BCI devices: Advances in implantable BCI technology hold promise for long-term neural recording and stimulation applications. Miniaturized implantable devices with wireless communication capabilities enable continuous monitoring of brain activity and delivery of therapeutic interventions, offering new opportunities for treating neurological disorders and enhancing cognitive function.
Integration with machine learning and artificial intelligence
-
Closed-loop BCI systems: Integration with machine learning and artificial intelligence enables the development of closed-loop BCI systems that adaptively modulate feedback and stimulation based on user performance and cognitive states. By incorporating adaptive algorithms, closed-loop BCIs can optimize task performance, enhance learning, and tailor interventions to individual user needs.
-
Brain-inspired computing: Researchers are exploring brain-inspired computing architectures and algorithms inspired by principles of neural computation. These bio-inspired approaches offer efficient and scalable solutions for processing and interpreting neural signals in real-time, opening new possibilities for energy-efficient and high-performance BCIs.
Potential societal impacts and ethical implications
-
Accessibility and inclusivity: As BCIs become more widespread and accessible, ensuring equitable access and inclusivity for individuals from diverse backgrounds and abilities is essential. Efforts to address barriers to access, such as cost, usability, and stigma, are crucial to maximizing the societal benefits of BCI technology and promoting social inclusion.
-
Ethical considerations: Ethical implications surrounding the use of BCIs, including privacy, consent, autonomy, and fairness, require careful consideration and proactive mitigation strategies. Ethical guidelines and regulatory frameworks are needed to address ethical challenges and ensure responsible development, deployment, and use of BCIs in research and applications.
-
Societal impacts: BCIs have the potential to reshape various aspects of society, including healthcare, education, communication, and entertainment. Understanding the broader societal impacts of BCIs, such as changes in social dynamics, employment patterns, and human-machine interactions, is essential for anticipating and addressing potential challenges and opportunities.
Conclusion
Recap of the importance and potential of BCIs
Throughout this discussion, we've explored the fascinating world of Brain-Computer Interfaces (BCIs) and their transformative potential across a wide range of applications. BCIs represent a groundbreaking technology that establishes direct communication pathways between the brain and external devices or computer systems, enabling individuals to control technology using their thoughts alone. From assisting individuals with disabilities to enhancing cognitive abilities and revolutionizing virtual experiences, BCIs offer unprecedented opportunities for improving human health, well-being, and quality of life.
Call to action for further research and development
While BCIs have made significant strides in recent years, there is still much to be explored and achieved. Continued research and development in BCI technology are essential to unlock its full potential and address existing challenges and limitations. This includes advancing neural decoding algorithms, improving the miniaturization and wearability of BCI devices, integrating BCIs with machine learning and artificial intelligence, and addressing ethical and societal implications. By fostering interdisciplinary collaboration, innovation, and investment in BCI research and development, we can accelerate progress and realize the promise of BCIs to transform healthcare, education, communication, and beyond.
Reflection on the transformative role of BCIs in neuroscience and technology
Looking ahead, BCIs have the potential to revolutionize our understanding of the brain and cognition, offering new insights into neural function, communication, and human-machine interaction. Beyond their practical applications, BCIs hold promise for advancing fundamental research in neuroscience, uncovering the mysteries of the mind, and unlocking new frontiers of knowledge and discovery. As we embark on this journey of exploration and innovation, let us embrace the transformative role of BCIs in shaping the future of neuroscience, technology, and society, and work together to harness their full potential for the benefit of all.