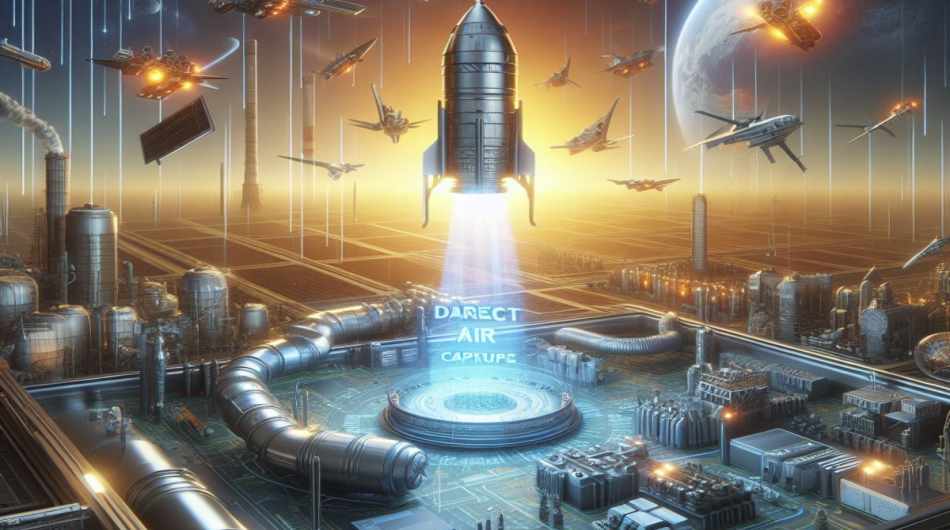
Direct air capture (DAC) technology is an emerging approach to mitigating climate change by removing carbon dioxide (CO₂) directly from the atmosphere. As the world grapples with the urgent need to reduce greenhouse gas emissions and limit global warming, DAC has gained increasing attention as a potential tool for achieving net-zero emissions and even net-negative emissions in the long term.
The Need for Carbon Dioxide Removal
The Earth's atmosphere has experienced a significant increase in CO₂ concentration since the beginning of the industrial era, primarily due to human activities such as burning fossil fuels, deforestation, and land-use changes. This increase in atmospheric CO₂ is the main driver of global warming and climate change, which pose severe threats to ecosystems, human health, and the global economy.
To limit global warming to well below 2°C above pre-industrial levels, as set out in the Paris Agreement, it is crucial to drastically reduce greenhouse gas emissions and achieve net-zero emissions by the middle of this century. However, many studies suggest that emission reductions alone may not be sufficient to meet this goal and that active removal of CO₂ from the atmosphere will also be necessary.
This is where carbon dioxide removal (CDR) technologies, such as direct air capture, come into play. By removing CO₂ directly from the atmosphere, DAC can complement emission reduction efforts and help offset emissions from hard-to-abate sectors, such as aviation and agriculture. Additionally, if deployed at a large scale, DAC has the potential to achieve net-negative emissions, effectively reversing some of the historical CO₂ emissions and helping to stabilize the Earth's climate.
Fundamentals of Direct Air Capture
Direct air capture is a process that involves the extraction of CO₂ directly from ambient air using chemical or physical sorbents. The captured CO₂ can then be either permanently stored in geological formations (a process known as carbon capture and storage, or CCS) or utilized in various applications, such as enhanced oil recovery, production of synthetic fuels, or as a feedstock for industrial processes (a process known as carbon capture and utilization, or CCU).
The basic principle behind DAC is to expose a sorbent material to air, which selectively binds with CO₂ molecules while allowing other components of air, such as nitrogen and oxygen, to pass through. The CO₂-laden sorbent is then regenerated by applying heat, pressure, or other means, releasing the captured CO₂ in a concentrated form. The regenerated sorbent can then be reused for another capture cycle.
Sorbent Materials
The choice of sorbent material is a critical factor in the design and performance of DAC systems. An ideal sorbent should have high selectivity for CO₂, high capacity for CO₂ capture, fast kinetics for adsorption and desorption, low regeneration energy requirements, and long-term stability and durability.
There are two main classes of sorbents used in DAC systems: solid sorbents and liquid sorbents.
Solid Sorbents
Solid sorbents are materials that capture CO₂ through physical adsorption or chemical absorption. Some common types of solid sorbents used in DAC include:
-
Zeolites: Porous aluminosilicate minerals with high surface area and selectivity for CO₂
-
Metal-organic frameworks (MOFs): Highly porous crystalline materials composed of metal ions and organic linkers, with tunable properties for CO₂ capture
-
Activated carbon: Porous carbon materials with high surface area and affinity for CO₂
-
Amine-functionalized sorbents: Solid materials, such as silica or polymers, that are functionalized with amine groups to enhance CO₂ capture through chemical absorption
Solid sorbents offer several advantages, such as high CO₂ selectivity, low energy requirements for regeneration, and the ability to operate at a wide range of temperatures and pressures. However, they also face challenges, such as limited CO₂ capacity, slow kinetics, and the need for frequent replacement or regeneration.
Liquid Sorbents
Liquid sorbents are solutions that capture CO₂ through chemical absorption. The most common type of liquid sorbent used in DAC is an aqueous solution of alkaline compounds, such as sodium hydroxide (NaOH) or potassium hydroxide (KOH). When exposed to air, these solutions react with CO₂ to form carbonates or bicarbonates, effectively capturing the CO₂ from the atmosphere.
Liquid sorbents offer several advantages over solid sorbents, such as higher CO₂ capacity, faster kinetics, and the ability to operate in a continuous flow process. However, they also face challenges, such as the need for large amounts of water, the potential for corrosion and degradation of equipment, and the high energy requirements for regeneration.
System Designs
There are several different designs and configurations of DAC systems, each with its advantages and challenges. Some of the most common designs include:
Passive Air Contactor Systems
Passive air contactor systems rely on natural airflow to bring CO₂ into contact with the sorbent material. These systems typically consist of large, stationary structures, such as towers or walls, that are coated with or filled with sorbent material. As air flows through the structure, CO₂ is captured by the sorbent, which is then periodically regenerated to release the concentrated CO₂.
Passive systems have the advantage of simplicity and low energy requirements, as they do not require active air circulation or temperature control. However, they also have limitations, such as low CO₂ capture rates, dependence on local wind conditions, and the need for large land areas.
Active Air Circulation Systems
Active air circulation systems use fans or blowers to actively circulate air through the sorbent material, allowing for higher CO₂ capture rates and more compact system designs. These systems can be further classified into two main types: packed bed systems and fluidized bed systems.
Packed bed systems consist of a container filled with the sorbent material, through which air is forced using a fan or blower. The CO₂-laden air passes through the sorbent bed, where the CO₂ is captured, and the purified air is released back into the atmosphere. The sorbent is then regenerated using heat or pressure, and the cycle repeats.
Fluidized bed systems use a stream of air or gas to suspend the sorbent particles in a fluidized state, creating a turbulent mixing zone that enhances contact between the sorbent and the CO₂-laden air. These systems can achieve higher CO₂ capture rates and more efficient sorbent utilization compared to packed bed systems, but they also require more complex designs and higher energy inputs.
Membrane-Based Systems
Membrane-based DAC systems use selective membranes to separate CO₂ from air. These membranes are typically made of polymeric or inorganic materials that allow CO₂ to permeate through while blocking other components of air. The CO₂-rich permeate stream is then further processed to obtain concentrated CO₂, while the CO₂-depleted air is released back into the atmosphere.
Membrane-based systems offer several advantages, such as high CO₂ selectivity, low energy requirements, and the ability to operate at a wide range of scales. However, they also face challenges, such as limited CO₂ permeability, the need for high membrane surface areas, and the potential for membrane fouling and degradation over time.
Challenges and Opportunities
Despite the promising potential of DAC technology, there are still several significant challenges that need to be addressed to enable its widespread deployment and impact on climate change mitigation. At the same time, DAC also presents numerous opportunities for innovation, economic growth, and environmental sustainability.
Technical Challenges
One of the main technical challenges facing DAC is the low concentration of CO₂ in ambient air, which is typically around 400 parts per million (ppm). This means that large volumes of air need to be processed to capture a significant amount of CO₂, requiring substantial energy inputs and infrastructure. Additionally, the presence of moisture, dust, and other contaminants in the air can interfere with the performance and durability of sorbent materials and system components.
Another challenge is the energy requirements for sorbent regeneration and CO₂ compression. The process of releasing the captured CO₂ from the sorbent and compressing it for transport and storage can be energy-intensive, particularly for solid sorbents that require high temperatures for regeneration. Developing more efficient and low-carbon energy sources for DAC, such as renewable electricity or waste heat, is crucial for ensuring the net carbon benefits of the technology.
Economic Challenges
The high cost of DAC systems is another major barrier to their widespread deployment. Current estimates of the cost of CO₂ capture using DAC range from $100 to $1,000 per tonne of CO₂, depending on the specific technology and system design. These costs are significantly higher than those of other CO₂ capture methods, such as point-source capture from industrial facilities, which can range from $50 to $100 per tonne of CO₂.
To make DAC economically viable, substantial cost reductions are needed through technological innovation, economies of scale, and supportive policies and incentives. Additionally, developing viable business models and markets for the captured CO₂, such as in enhanced oil recovery or the production of low-carbon fuels and materials, can help offset the costs of DAC and create additional revenue streams.
Environmental and Social Challenges
While DAC has the potential to provide significant environmental benefits by removing CO₂ from the atmosphere, it also presents some environmental and social challenges that need to be carefully considered and addressed.
One concern is the potential land-use impacts of large-scale DAC deployment, particularly for passive air contactor systems that require large surface areas. The siting of DAC facilities needs to take into account factors such as land availability, proximity to CO₂ storage sites or utilization opportunities, and potential impacts on local ecosystems and communities.
Another challenge is the potential for DAC to create a "moral hazard" by reducing the urgency and incentives for emission reductions in other sectors. It is crucial to emphasize that DAC is not a silver bullet for climate change mitigation and that it must be pursued in parallel with aggressive efforts to reduce emissions from fossil fuels and other sources.
Opportunities for Innovation and Growth
Despite these challenges, DAC also presents significant opportunities for technological innovation, economic growth, and environmental sustainability.
The development and deployment of DAC systems can create new industries and jobs in areas such as materials science, chemical engineering, and renewable energy. It can also stimulate innovation in related fields, such as CO₂ utilization and low-carbon manufacturing, creating additional economic opportunities and environmental benefits.
DAC can also play a crucial role in achieving net-zero and net-negative emissions targets, particularly in hard-to-abate sectors such as aviation and agriculture. By providing a means to offset residual emissions and even remove historical emissions from the atmosphere, DAC can help balance the global carbon budget and limit the risks and impacts of climate change.
Furthermore, DAC can provide a new source of low-carbon CO₂ for various industrial and commercial applications, such as in the production of synthetic fuels, plastics, and building materials. This can help reduce reliance on fossil-based feedstocks and create new markets for sustainable products and services.
Current State and Future Prospects
The field of direct air capture is still in its early stages, with only a handful of pilot and demonstration projects currently in operation worldwide. However, there is growing interest and investment in DAC from both the public and private sectors, driven by the urgent need for climate change mitigation and the potential economic and environmental benefits of the technology.
Pilot and Demonstration Projects
Some notable examples of current DAC projects include:
-
Climeworks: A Swiss company that operates several DAC plants in Europe, including a pilot plant in Italy that captures up to 150 tonnes of CO₂ per year using solid sorbent technology.
-
Carbon Engineering: A Canadian company that operates a pilot plant in British Columbia, Canada, which captures up to 1 tonne of CO₂ per day using a liquid sorbent system. The company is also developing a commercial-scale DAC plant in partnership with Occidental Petroleum.
-
Global Thermostat: A U.S. company that operates a pilot plant in Alabama, USA, which captures up to 4,000 tonnes of CO₂ per year using a solid sorbent system.
-
Prometheus Fuels: A U.S. startup that is developing a novel DAC technology that uses electrochemical cells to capture CO₂ directly from the air and convert it into synthetic fuels.
These and other pilot projects are providing valuable data and experience on the technical and economic performance of different DAC technologies and system designs, helping to identify challenges and opportunities for further development and scale-up.
Policy and Investment Landscape
In recent years, there has been growing policy and investment support for DAC and other carbon dioxide removal technologies, reflecting the increasing recognition of their potential role in climate change mitigation.
Several countries, including the United States, the United Kingdom, and Norway, have established funding programs and incentives for DAC research, development, and deployment. For example, the U.S. Department of Energy has launched a $22 million funding opportunity for DAC technologies, while the UK government has committed £100 million to support the development of greenhouse gas removal technologies, including DAC.
Private sector investment in DAC is also increasing, with several major corporations and investors announcing plans to fund or develop DAC projects. For example, Microsoft has pledged to invest $1 billion in carbon removal technologies, including DAC, while Elon Musk has launched a $100 million prize for the development of scalable carbon capture and storage solutions.
However, the current level of funding and investment in DAC is still relatively small compared to the scale of the climate challenge and the potential of the technology. Substantially greater public and private investment will be needed to accelerate the development and deployment of DAC and other carbon removal solutions in the coming decades.
Future Scenarios and Roadmaps
Several studies and roadmaps have explored the potential future deployment and impact of DAC technology under different climate change mitigation scenarios.
For example, the International Energy Agency (IEA) has developed a Sustainable Development Scenario (SDS) that envisions a rapid scale-up of DAC and other carbon removal technologies to help limit global warming to well below 2°C. Under this scenario, DAC could capture up to 10 gigatonnes (Gt) of CO₂ per year by 2070, equivalent to roughly one-quarter of current annual global CO₂ emissions.
Similarly, a study by the National Academies of Sciences, Engineering, and Medicine (NASEM) in the United States has developed a research agenda and deployment strategy for negative emissions technologies (NETs), including DAC. The study estimates that NETs could remove up to 10 Gt of CO₂ per year by 2050 and up to 20 Gt per year by 2100, with DAC playing a significant role in this deployment.
However, achieving these ambitious deployment levels will require substantial efforts to address the technical, economic, and policy challenges facing DAC and other carbon removal technologies. This will likely involve a combination of sustained research and development, supportive policies and incentives, and international cooperation and coordination.
Conclusion
Direct air capture technology represents a promising tool for mitigating climate change by removing CO₂ directly from the atmosphere. By complementing emission reduction efforts and enabling net-negative emissions in the long term, DAC can play a crucial role in achieving the goals of the Paris Agreement and limiting the risks and impacts of global warming.
However, realizing the full potential of DAC will require overcoming significant technical, economic, and social challenges, from reducing the costs and energy requirements of CO₂ capture to ensuring the environmental and social sustainability of large-scale deployment.
At the same time, DAC presents significant opportunities for technological innovation, economic growth, and environmental sustainability. The development and deployment of DAC systems can create new industries and jobs, stimulate innovation in related fields, and provide a new source of low-carbon CO₂ for various applications.
To seize these opportunities and address the challenges, greater public and private investment in DAC research, development, and deployment is needed, along with supportive policies and incentives at the national and international levels. This will require a collaborative and multidisciplinary approach, involving scientists, engineers, policymakers, and stakeholders from across society.
As the world grapples with the urgent need to address climate change, direct air capture technology offers a promising pathway towards a more sustainable and resilient future. By investing in DAC and other carbon removal solutions, we can help ensure a stable climate and a thriving planet for generations to come.