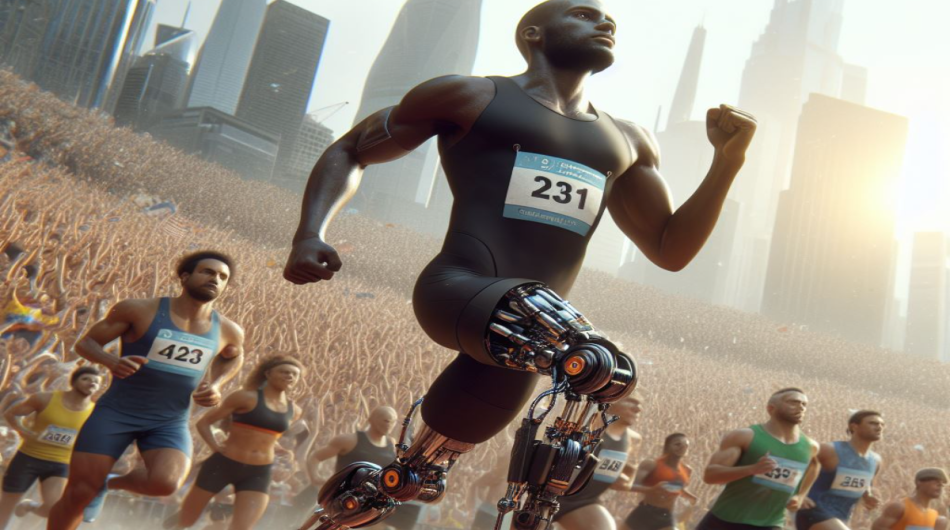
Advanced bionics is a rapidly evolving field that combines the principles of biology, engineering, and technology to develop innovative solutions for a wide range of medical and rehabilitative applications. This interdisciplinary approach aims to create devices and systems that can integrate with or mimic biological systems, enhancing or restoring lost functions and improving the quality of life for individuals with various conditions or disabilities.
Applications of Advanced Bionics
Advanced Bionics has the potential to revolutionize the way we treat and manage a wide range of medical conditions and disabilities. Some of the most prominent applications of advanced bionics include:
Prosthetics and Exoskeletons
One of the most well-known applications of advanced bionics is in the development of prosthetic limbs and exoskeletons. These devices are designed to replace or augment missing or impaired body parts, enabling individuals with amputations or mobility impairments to regain function and independence. Advanced bionic prosthetics incorporate a range of technologies, such as myoelectric control, sensory feedback, and osseointegration, to provide users with more natural and intuitive control over their artificial limbs. Exoskeletons, on the other hand, are wearable robotic devices that can enhance the strength, endurance, and mobility of the user, with potential applications in rehabilitation, industrial settings, and military use.
Sensory Restoration
Advanced Bionics also plays a crucial role in restoring sensory functions, such as hearing and vision, for individuals with sensory impairments. Cochlear implants, for example, are bionic devices that bypass damaged parts of the inner ear and directly stimulate the auditory nerve, allowing individuals with severe to profound hearing loss to perceive sound. Similarly, retinal implants and optogenetic therapies are being developed to restore visual function in individuals with blindness or low vision by stimulating the remaining healthy cells in the retina or directly activating the visual cortex.
Neural Interfaces
Neural interfaces are another key application area for advanced bionics, aiming to establish direct communication pathways between the nervous system and external devices. These interfaces can be used for a variety of purposes, such as controlling prosthetic limbs, restoring sensory feedback, and treating neurological disorders. Some examples of neural interface technologies include brain-computer interfaces (BCIs), which allow users to control computers or other devices using their brain activity; peripheral nerve interfaces, which stimulate or record from peripheral nerves to control prosthetics or treat chronic pain; and deep brain stimulation (DBS), which involves implanting electrodes in specific regions of the brain to treat conditions such as Parkinson's disease, essential tremor, and obsessive-compulsive disorder.
Tissue Engineering and Regenerative Medicine
Advanced Bionics also intersects with the fields of tissue engineering and regenerative medicine, which aim to repair, replace, or regenerate damaged or diseased tissues and organs. Bionic technologies can be used to create scaffolds and biomaterials that guide the growth and differentiation of cells, promoting the regeneration of functional tissues. Additionally, advanced bionic devices, such as bioartificial organs and lab-on-a-chip systems, can be used to support or replace the function of damaged organs, such as the liver, kidney, or pancreas, while waiting for a suitable transplant or as a long-term alternative to transplantation.
Technologies and Materials in Advanced Bionics
The development of advanced bionic devices and systems relies on a wide range of cutting-edge technologies and materials. Some of the key technologies and materials used in advanced bionics include:
Sensors and Actuators
Sensors and actuators are essential components of many advanced bionic devices, enabling them to interact with the biological system and the environment. Sensors are used to detect and measure various physical, chemical, and biological signals, such as pressure, temperature, pH, and electrical activity. Some common types of sensors used in advanced bionics include electromyographic (EMG) sensors for detecting muscle activity, force and pressure sensors for monitoring gait and balance, and biochemical sensors for measuring metabolite or neurotransmitter levels. Actuators, on the other hand, are responsible for generating movement or other forms of output in response to control signals. Examples of actuators used in advanced bionics include electric motors, piezoelectric materials, shape memory alloys, and pneumatic or hydraulic systems.
Biomaterials and Coatings
Biomaterials are another crucial aspect of advanced bionics, as they are used to construct the interfaces between the bionic device and the biological system. These materials must be biocompatible, meaning they should not elicit an adverse immune response or cause toxicity, and they should also have appropriate mechanical, electrical, and chemical properties for their intended application. Some common biomaterials used in advanced bionics include metals, such as titanium and stainless steel, for their strength and durability; polymers, such as silicone and polyurethane, for their flexibility and ease of fabrication; and ceramics, such as hydroxyapatite and zirconia, for their hardness and bioactivity. Additionally, various coatings and surface modifications can be applied to biomaterials to improve their biocompatibility, reduce inflammation, and promote tissue integration.
Power and Energy Management
Power and energy management are critical considerations in the design of advanced bionic devices, as these systems often need to operate continuously or for extended periods without requiring frequent battery replacements or recharging. Some strategies for power and energy management in advanced bionics include the use of high-density batteries, such as lithium-ion or lithium-polymer batteries; energy harvesting techniques, such as piezoelectric or thermoelectric generators that convert movement or heat into electrical energy; and wireless power transfer methods, such as inductive coupling or ultrasonic energy transfer, which allow for the non-invasive charging of implanted devices.
Data Processing and Machine Learning
As advanced bionic devices become more sophisticated and capable of collecting and analyzing large amounts of data, the incorporation of data processing and machine learning techniques becomes increasingly important. These technologies can be used to extract meaningful information from complex biological signals, adapt the behaviour of the bionic device to the user's needs and preferences, and enable more natural and intuitive control of the system. Some examples of data processing and machine learning approaches used in advanced bionics include signal filtering and feature extraction techniques, such as wavelet analysis and independent component analysis, for isolating relevant information from noisy biological signals; pattern recognition and classification algorithms, such as support vector machines and artificial neural networks, for identifying specific user intentions or motor commands; and reinforcement learning and adaptive control methods, such as actor-critic models and Bayesian optimization, for continuously updating and improving the performance of the bionic device based on user feedback and experience.
Challenges and Future Prospects
Despite the significant advancements made in the field of advanced bionics, there are still several challenges that need to be addressed to fully realize the potential of these technologies. Some of the key challenges and prospects for advanced bionics include:
Biocompatibility and Long-term Stability
One of the main challenges in advanced bionics is ensuring the biocompatibility and long-term stability of the devices and materials used. Bionic devices that are implanted or come into direct contact with biological tissues must be designed to minimize the risk of infection, inflammation, and other adverse reactions. Additionally, these devices must be able to withstand the harsh and dynamic environment of the human body, including exposure to various chemicals, mechanical stresses, and temperature fluctuations, without degrading or losing functionality over time. To address these challenges, researchers are exploring the use of novel biomaterials, such as self-healing polymers and bioresorbable metals, as well as advanced surface modification techniques, such as plasma treatment and biomolecule immobilization, to improve the biocompatibility and durability of bionic devices.
Seamless Integration and Control
Another major challenge in advanced bionics is achieving seamless integration and control of the devices with the user's body and intentions. For bionic devices to be truly effective and accepted by users, they must be able to function as natural extensions of the body, providing intuitive and responsive control without requiring extensive training or cognitive effort. This requires the development of advanced sensing and actuation technologies that can accurately detect and interpret the user's motor commands and sensory feedback, as well as adaptive control algorithms that can learn and adapt to the user's individual needs and preferences over time. Additionally, researchers are exploring the use of novel neural interface techniques, such as optogenetics and magnetogenetics, which allow for the precise and selective stimulation or recording of specific neural populations, potentially enabling more natural and finely tuned control of bionic devices.
Ethical and Regulatory Considerations
As advanced bionic technologies become more sophisticated and widely available, there are also important ethical and regulatory considerations that need to be addressed. Some of the key ethical concerns surrounding advanced bionics include issues of privacy and data security, as these devices often collect and transmit sensitive personal and health information; equity and access, as the high cost and limited availability of some bionic technologies, may create disparities in who can benefit from them; and identity and autonomy, as the integration of bionic devices with the human body and mind may raise questions about the boundaries of the self and the extent to which individuals have control over their bodies and abilities. Additionally, the development and deployment of advanced bionic technologies are subject to various regulatory requirements and oversight, including safety and efficacy testing, manufacturing standards, and post-market surveillance, which can create significant barriers and delays in bringing these technologies to market.
Conclusion
Advanced bionics represents a transformative field that has the potential to revolutionize the way we treat and manage a wide range of medical conditions and disabilities, from sensory impairments and neurological disorders to limb loss and organ failure. By combining the principles of biology, engineering, and technology, researchers and innovators are developing sophisticated devices and systems that can integrate with or mimic biological systems, restoring or enhancing lost functions and improving the quality of life for countless individuals.
The development of advanced bionic technologies relies on a multidisciplinary approach, drawing on expertise from fields such as materials science, sensors and actuators, power and energy management, and data processing and machine learning. While there are still significant challenges to be addressed, including issues of biocompatibility and long-term stability, seamless integration and control, and ethical and regulatory considerations, the prospects for advanced bionics are truly exciting.
As research and innovation in this field continue to progress, we can expect to see the development of increasingly sophisticated and personalized bionic devices that can adapt to the unique needs and preferences of each user. From fully implantable and autonomous sensory prosthetics to regenerative bionic scaffolds and bioartificial organs, the possibilities for advanced bionics are virtually limitless.
Ultimately, the success of advanced bionics will depend not only on technological advancements but also on the active engagement and collaboration of all stakeholders, including researchers, clinicians, policymakers, and most importantly, the individuals and communities who stand to benefit from these life-changing technologies. By working together to address the technical, ethical, and societal challenges surrounding advanced bionics, we can unlock the full potential of this field and create a future in which every individual has the opportunity to live a full and fulfilling life, regardless of their physical or sensory abilities.